I Thought We’d Learned Nothing From the Pandemic. I Wasn’t Seeing the Full Picture
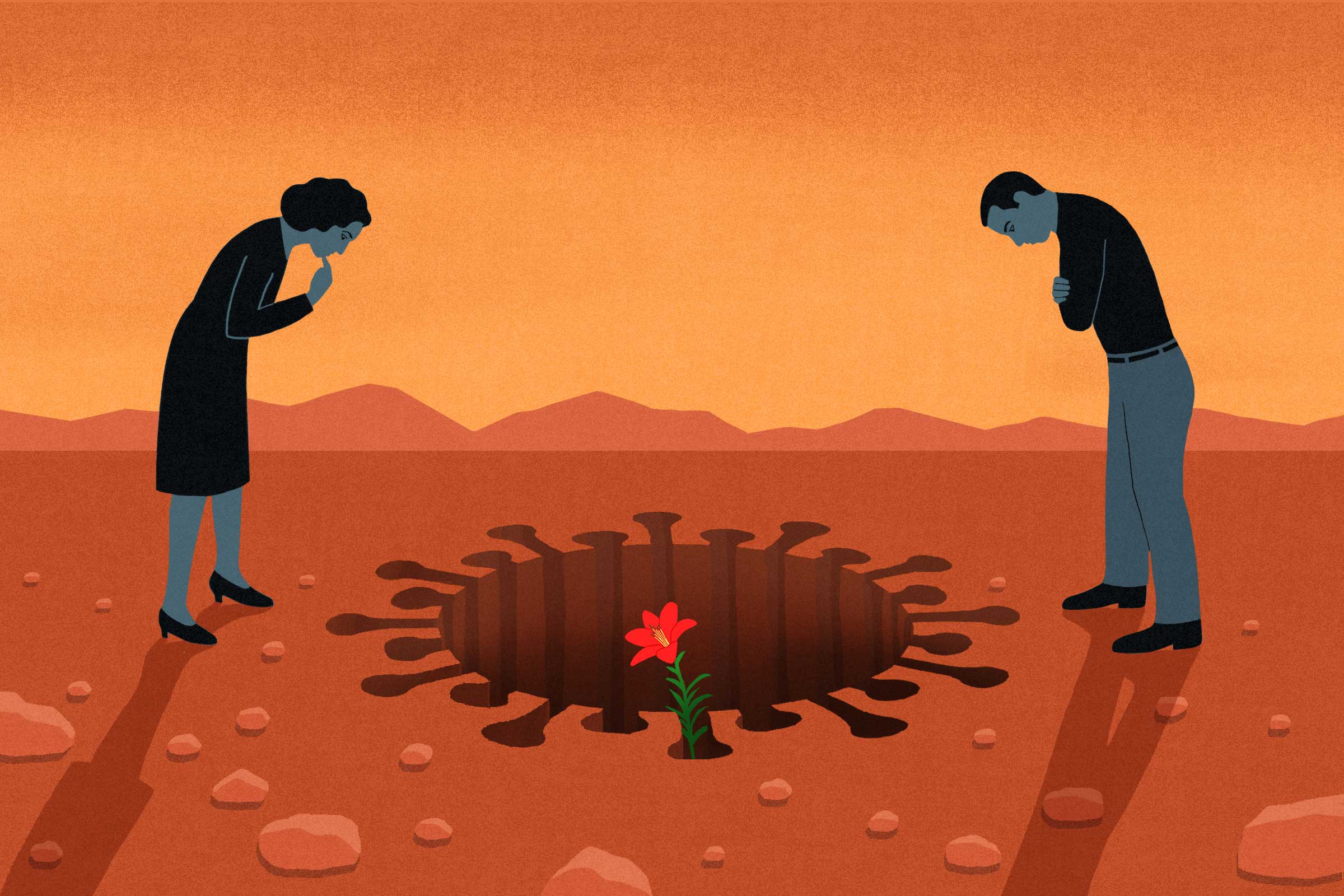
M y first home had a back door that opened to a concrete patio with a giant crack down the middle. When my sister and I played, I made sure to stay on the same side of the divide as her, just in case. The 1988 film The Land Before Time was one of the first movies I ever saw, and the image of the earth splintering into pieces planted its roots in my brain. I believed that, even in my own backyard, I could easily become the tiny Triceratops separated from her family, on the other side of the chasm, as everything crumbled into chaos.
Some 30 years later, I marvel at the eerie, unexpected ways that cartoonish nightmare came to life – not just for me and my family, but for all of us. The landscape was already covered in fissures well before COVID-19 made its way across the planet, but the pandemic applied pressure, and the cracks broke wide open, separating us from each other physically and ideologically. Under the weight of the crisis, we scattered and landed on such different patches of earth we could barely see each other’s faces, even when we squinted. We disagreed viciously with each other, about how to respond, but also about what was true.
Recently, someone asked me if we’ve learned anything from the pandemic, and my first thought was a flat no. Nothing. There was a time when I thought it would be the very thing to draw us together and catapult us – as a capital “S” Society – into a kinder future. It’s surreal to remember those early days when people rallied together, sewing masks for health care workers during critical shortages and gathering on balconies in cities from Dallas to New York City to clap and sing songs like “Yellow Submarine.” It felt like a giant lightning bolt shot across the sky, and for one breath, we all saw something that had been hidden in the dark – the inherent vulnerability in being human or maybe our inescapable connectedness .

More from TIME
Read More: The Family Time the Pandemic Stole
But it turns out, it was just a flash. The goodwill vanished as quickly as it appeared. A couple of years later, people feel lied to, abandoned, and all on their own. I’ve felt my own curiosity shrinking, my willingness to reach out waning , my ability to keep my hands open dwindling. I look out across the landscape and see selfishness and rage, burnt earth and so many dead bodies. Game over. We lost. And if we’ve already lost, why try?
Still, the question kept nagging me. I wondered, am I seeing the full picture? What happens when we focus not on the collective society but at one face, one story at a time? I’m not asking for a bow to minimize the suffering – a pretty flourish to put on top and make the whole thing “worth it.” Yuck. That’s not what we need. But I wondered about deep, quiet growth. The kind we feel in our bodies, relationships, homes, places of work, neighborhoods.
Like a walkie-talkie message sent to my allies on the ground, I posted a call on my Instagram. What do you see? What do you hear? What feels possible? Is there life out here? Sprouting up among the rubble? I heard human voices calling back – reports of life, personal and specific. I heard one story at a time – stories of grief and distrust, fury and disappointment. Also gratitude. Discovery. Determination.
Among the most prevalent were the stories of self-revelation. Almost as if machines were given the chance to live as humans, people described blossoming into fuller selves. They listened to their bodies’ cues, recognized their desires and comforts, tuned into their gut instincts, and honored the intuition they hadn’t realized belonged to them. Alex, a writer and fellow disabled parent, found the freedom to explore a fuller version of herself in the privacy the pandemic provided. “The way I dress, the way I love, and the way I carry myself have both shrunk and expanded,” she shared. “I don’t love myself very well with an audience.” Without the daily ritual of trying to pass as “normal” in public, Tamar, a queer mom in the Netherlands, realized she’s autistic. “I think the pandemic helped me to recognize the mask,” she wrote. “Not that unmasking is easy now. But at least I know it’s there.” In a time of widespread suffering that none of us could solve on our own, many tended to our internal wounds and misalignments, large and small, and found clarity.
Read More: A Tool for Staying Grounded in This Era of Constant Uncertainty
I wonder if this flourishing of self-awareness is at least partially responsible for the life alterations people pursued. The pandemic broke open our personal notions of work and pushed us to reevaluate things like time and money. Lucy, a disabled writer in the U.K., made the hard decision to leave her job as a journalist covering Westminster to write freelance about her beloved disability community. “This work feels important in a way nothing else has ever felt,” she wrote. “I don’t think I’d have realized this was what I should be doing without the pandemic.” And she wasn’t alone – many people changed jobs , moved, learned new skills and hobbies, became politically engaged.
Perhaps more than any other shifts, people described a significant reassessment of their relationships. They set boundaries, said no, had challenging conversations. They also reconnected, fell in love, and learned to trust. Jeanne, a quilter in Indiana, got to know relatives she wouldn’t have connected with if lockdowns hadn’t prompted weekly family Zooms. “We are all over the map as regards to our belief systems,” she emphasized, “but it is possible to love people you don’t see eye to eye with on every issue.” Anna, an anti-violence advocate in Maine, learned she could trust her new marriage: “Life was not a honeymoon. But we still chose to turn to each other with kindness and curiosity.” So many bonds forged and broken, strengthened and strained.
Instead of relying on default relationships or institutional structures, widespread recalibrations allowed for going off script and fortifying smaller communities. Mara from Idyllwild, Calif., described the tangible plan for care enacted in her town. “We started a mutual-aid group at the beginning of the pandemic,” she wrote, “and it grew so quickly before we knew it we were feeding 400 of the 4000 residents.” She didn’t pretend the conditions were ideal. In fact, she expressed immense frustration with our collective response to the pandemic. Even so, the local group rallied and continues to offer assistance to their community with help from donations and volunteers (many of whom were originally on the receiving end of support). “I’ve learned that people thrive when they feel their connection to others,” she wrote. Clare, a teacher from the U.K., voiced similar conviction as she described a giant scarf she’s woven out of ribbons, each representing a single person. The scarf is “a collection of stories, moments and wisdom we are sharing with each other,” she wrote. It now stretches well over 1,000 feet.
A few hours into reading the comments, I lay back on my bed, phone held against my chest. The room was quiet, but my internal world was lighting up with firefly flickers. What felt different? Surely part of it was receiving personal accounts of deep-rooted growth. And also, there was something to the mere act of asking and listening. Maybe it connected me to humans before battle cries. Maybe it was the chance to be in conversation with others who were also trying to understand – what is happening to us? Underneath it all, an undeniable thread remained; I saw people peering into the mess and narrating their findings onto the shared frequency. Every comment was like a flare into the sky. I’m here! And if the sky is full of flares, we aren’t alone.
I recognized my own pandemic discoveries – some minor, others massive. Like washing off thick eyeliner and mascara every night is more effort than it’s worth; I can transform the mundane into the magical with a bedsheet, a movie projector, and twinkle lights; my paralyzed body can mother an infant in ways I’d never seen modeled for me. I remembered disappointing, bewildering conversations within my own family of origin and our imperfect attempts to remain close while also seeing things so differently. I realized that every time I get the weekly invite to my virtual “Find the Mumsies” call, with a tiny group of moms living hundreds of miles apart, I’m being welcomed into a pocket of unexpected community. Even though we’ve never been in one room all together, I’ve felt an uncommon kind of solace in their now-familiar faces.
Hope is a slippery thing. I desperately want to hold onto it, but everywhere I look there are real, weighty reasons to despair. The pandemic marks a stretch on the timeline that tangles with a teetering democracy, a deteriorating planet , the loss of human rights that once felt unshakable . When the world is falling apart Land Before Time style, it can feel trite, sniffing out the beauty – useless, firing off flares to anyone looking for signs of life. But, while I’m under no delusions that if we just keep trudging forward we’ll find our own oasis of waterfalls and grassy meadows glistening in the sunshine beneath a heavenly chorus, I wonder if trivializing small acts of beauty, connection, and hope actually cuts us off from resources essential to our survival. The group of abandoned dinosaurs were keeping each other alive and making each other laugh well before they made it to their fantasy ending.
Read More: How Ice Cream Became My Own Personal Act of Resistance
After the monarch butterfly went on the endangered-species list, my friend and fellow writer Hannah Soyer sent me wildflower seeds to plant in my yard. A simple act of big hope – that I will actually plant them, that they will grow, that a monarch butterfly will receive nourishment from whatever blossoms are able to push their way through the dirt. There are so many ways that could fail. But maybe the outcome wasn’t exactly the point. Maybe hope is the dogged insistence – the stubborn defiance – to continue cultivating moments of beauty regardless. There is value in the planting apart from the harvest.
I can’t point out a single collective lesson from the pandemic. It’s hard to see any great “we.” Still, I see the faces in my moms’ group, making pancakes for their kids and popping on between strings of meetings while we try to figure out how to raise these small people in this chaotic world. I think of my friends on Instagram tending to the selves they discovered when no one was watching and the scarf of ribbons stretching the length of more than three football fields. I remember my family of three, holding hands on the way up the ramp to the library. These bits of growth and rings of support might not be loud or right on the surface, but that’s not the same thing as nothing. If we only cared about the bottom-line defeats or sweeping successes of the big picture, we’d never plant flowers at all.
More Must-Reads from TIME
- How the Electoral College Actually Works
- Your Vote Is Safe
- Mel Robbins Will Make You Do It
- Why Vinegar Is So Good for You
- The Surprising Health Benefits of Pain
- You Don’t Have to Dread the End of Daylight Saving
- The 20 Best Halloween TV Episodes of All Time
- Meet TIME's Newest Class of Next Generation Leaders
Contact us at [email protected]
How to Write About Coronavirus / COVID-19 In Your College Essay & Application
TABLE OF CONTENTS
Option 2: the slightly more creative way, how to use narrative structure to describe your pandemic experience if you want it to be all of your essay, should i write about coronavirus/ covid-19 in my college essay.
This year, the Common App is including a special 250-word section allowing students to describe the impacts of COVID-19 on their lives. Here’s the official word from the Common App website:
We want to provide colleges with the information they need, with the goal of having students answer COVID-19 questions only once while using the rest of the application as they would have before to share their interests and perspectives beyond COVID-19.
Below is the question applicants will see:
Community disruptions such as COVID-19 and natural disasters can have deep and long-lasting impacts. If you need it, this space is yours to describe those impacts. Colleges care about the effects on your health and well-being, safety, family circumstances, future plans, and education, including access to reliable technology and quiet study spaces.
Do you wish to share anything on this topic? Y/N
Please use this space to describe how these events have impacted you.
The question will be optional and will appear in the Additional Information section of the application. The response length will be limited to 250 words.
It’s worth reading from the Common App website directly , which also notes that counselors will have 500 words to detail impacts that their schools have experienced based on the pandemic.
Note: If you’re applying via the Coalition Application, you’ll also have an opportunity to add information. Learn the details here . And the advice below will apply!
So should you write about coronavirus?
On this webinar at the 14:00 mark, I asked Rick Clark, Director of Admission at Georgia Tech, this very question. His response: “How could they not!” In other words: You totally have permission to write about this.
But it also kinda’ depends on your pandemic experience.
Which of the following best describes what the pandemic has been like for you?
It’s been okay . Online school wasn’t as good as real school, but I adapted, played video games maybe a little more than usual (so my sleeping schedule was weird), and hung out with my family a little more. TBH, though, things haven’t changed (or didn’t change) too much for me.
Very negative. Coronavirus rocked my world—and not in a good way. The pandemic has led to serious hardships for my family and me. It’s been incredibly stressful, and we’re still living with some uncertainty.
Very positive. And I feel weird saying that because I know so many people have been negatively impacted. But I’ve learned some new things/felt inspired/maybe even started a new project and (maybe even) I’ve even shifted the way I’m thinking about my future!
It’s been a roller coaster (so 2 and 3). In some ways, it’s been really difficult, but in other ways, it’s been productive, and I’m learning a lot.
Do a quick self-scan: Which feels most like your experience?
And if your experience has been way too complex to fit into one of these (admittedly) overly-simplistic and reductive categories, read on.
But based on these broad categories, I’d say ...
If your life hasn’t changed too much (so A), no need to write about it.
If the pandemic has been either very negative (B), very positive (C), or like a roller coaster (D), maybe do write about it.
The questions are: Where should you write about it in your application, and how?
You’ve got options.
Where to Write About COVID-19/ Coronavirus on Your College Application?
If the school you’re applying to is on the Common App, your options include:
The special COVID-19 question (250 words)
The Additional Information section (650 words)
Your main personal statement (650 words)
Below, I’ll offer tips on how to write each one. And if the school you’re applying to is not on the Common App, check out that school’s particular application.
There’s an old saying in the musical theater world that goes something like this: If what you want to say is so important that mere words can’t capture it, you sing it. And if not even singing can capture those feelings, you dance it. (More commonly: “If you can’t say it, sing it. If you can’t sing it, dance it.”)
That’s pretty much my advice when it comes to writing about coronavirus/COVID-19 on your college application.
How to Write About COVID-19/ Coronavirus on Your College Application
More specifically, if you feel as though you need to address your pandemic experience in your college application, I’d say:
If you can fit your pandemic experiences into the 250-word COVID-19 section, do it.
If you need more space, use the 650-word Additional Information section.
If a) your pandemic experience was one of the most important things that’s ever happened to you and there’s no way it could fit in both of the sections named above, b) you believe that describing your pandemic experience is the best way to demonstrate the values/skills/qualities that you’ll bring with you to a college campus, and c) you’ve spent at least an hour exploring other topics using high-quality brainstorming exercises with a partner and still haven’t come up with any other ideas, then you might consider using the 650-word personal statement.
But keep in mind that if you do write about the pandemic in your personal statement, then you can’t use the 250-word COVID-specific section for anything else. On the other hand, if you write about the pandemic in the COVID-specific space, you can use your personal statement for …. whatever you want.
Also note that the Common App is kind of hinting that you should use the 250-word COVID-19 section so that you can use the rest of your application for other things: “ We want to provide colleges with the information they need, with the goal of having students answer COVID-19 questions only once while using the rest of the application as they would have before to share their interests and perspectives beyond COVID-19.”
Again, I’m going to show you how to write something in each section, but can you sense which way I’m nudging you?
And btw, if you’re unsure what else to write your personal statement on, keep reading—I’ll share brainstorming exercises below.
How to Write About Coronavirus Using the Special COVID-19 (250-Word) Section on the Common App
Here again is the question you’ll see on the Common App:
Option 1: The Straightforward Way
If you did face significant challenges during the pandemic, one way to write about your pandemic experience is by using this structure:
a) Challenges Faced + Impacts on Me
b) What I Did about It
c) What I Learned
Below is an example of what this might look like. (It’s not an actual example, but was written by a former student to illustrate how you can write yours)
Example 1 (faced significant challenges):
Living in rural North Carolina, I have limited access to a consistent, high-speed internet connection. During the pandemic, my family did not have the means to upgrade to a higher internet speed and were working hard around the house trying to make ends meet. This meant I was often unable to access the internet in time to turn in assignments when they were due. It was also difficult to concentrate because our house is very small and everyone was working in close proximity.
Although I found it hard to focus on schoolwork, I communicated these problems to my family so that we could work together. I organized a rotating schedule for my parents and my older sister. We marked off the blocks of time during which we would need to be online and created a system in which only two family members would be on the internet at the same time. The people who were not busy would stay quiet indoors or go outside to talk. This made it so that the internet was faster and there was less noise inside the house.
I am proud to say that I used what I had at my disposal to make the best out of a difficult situation. The unusual conditions instilled in me the value of organization and clear communication. I found ways of adapting my work to fit new time constraints and will bring this knowledge with me to college. (240 words)
Quick Notes + Tips:
This kind of straightforward and factual tone is fine. In fact, some readers will prefer it.
Notice how the example above devotes one bullet point to each of the elements I mentioned: a) Challenges Faced + Impacts on Me, b) What I Did about It, c) What I Learned. And yes, bullet points are OK in this section.
Notice how, in the third bullet point, the author demonstrates a few values that will serve them in college and beyond: adaptability, organization, communication. For a list of values, click here .
Example 2 (did not face significant challenges):
I live in Marfa, Texas, where an important part of the local economy is the restaurant industry. Many businesses in the area were forced to shut down or operate in a more limited capacity. To support these people and their contributions to our community, I started an online blog to write reviews about the takeout my family and I ate during quarantine. I made sure to include details about how food could be ordered and what options they had for different dietary restrictions. As someone who has a very restrictive diet, I understand the importance of finding food that is healthy, delicious, and conscious of different dietary needs. I also wanted to encourage people to support their local businesses.
In addition, to keep myself physically active despite limited mobility, I created a makeshift gym in my house. I fashioned “dumbbells” out of old milk cartons filled with dirt and took an online class about weight training to build a balanced workout schedule. I even got my parents to join me once a week!
I stayed connected with friends during weekly sessions on Zoom and Discord. We often spent hours playing online board games like Bananagrams and Codenames. Because I enjoyed bonding over these games and being intellectually stimulated by the puzzles they posed, I ended up taking an online course in Python and am working on coding my first video game. (231 words)
Quick Notes + Tips:
Notice how in this example, which is not a real example either but was written for illustrative purposes, the author chooses three specific aspects of the pandemic and devotes one bullet point to each.
The author begins by describing a particular need (supporting local businesses) and what they did about it (started a blog). This demonstrates the values of leadership and entrepreneurialism—even without naming the values explicitly, which is fine.
The second bullet point addresses a separate value (health), and the author gives evidence that the impact went beyond themselves—to their parents!
Finally, the author demonstrates the value of what I like to call “curiosity with legs” (i.e., being interested in something—then doing something about it). Again, the tone is straightforward, which works well.
If this kind of straightforward, factual tone isn’t your thing, you could start with something that grabs our attention. Like this:
Example 3 (faced significant challenges):
“Jose, turn down the TV. MOM, THE STOVE WON’T TURN ON! Be quiet, I’m on a call. Zuli, have you seen the scissors anywhere?!” Life in quarantine was actually four lives squished together. The pandemic forced my parents, my older sister, and I into a space that wasn’t built for all of our preferences and professions. Living in a small, one-bedroom apartment in Los Angeles meant that internet speed was often slow and privacy was minimal. We were constantly yelling, stepping on each other’s toes. Although I was discouraged, I knew that the tension in our house came mostly from fear of uncertainty, not a lack of love. I kept a level head and called a family meeting. Together, we organized a rotating schedule, marking off when we needed to be online. With this information, we created a system in which only two family members would be on the internet at any time. The people who were not busy would keep quiet or go outside to talk. In a matter of days, the internet was faster and the noise had gone down. I did what I could to make the best out of a difficult situation. The unusual conditions instilled in me the value of organization and clear communication. And I learned how to hit pause when things got intense. We’re still squished, but our love is louder than our yelling. (231 words)
Quick Notes + Tips:
Using a slightly more creative approach is also fine. In fact, some readers may find it refreshing. But note that the “slightly more creative” opening is really just 24 words long. The rest is pretty straightforward. So don’t spend too much time obsessing over this. The information you share is what’s most important here.
Notice how, in this example, the author takes the framework of the first example essay and bends it into a more cohesive narrative. It still follows the same path (i.e., identifying the challenges/effects, how you dealt with them, what you learned), but smooths the edges between those sections. It also gets a bit more creative with the intro, hooking readers with some intriguing sentence fragments. If you want to do something a little unconventional, this is a good framework you can use.
Also notice that the author still keeps all the relevant information here. It’s still crucial for her to communicate that the internet speed was slow and the house was crowded because that’s essentially the answer to the prompt. Those are challenges that will help admissions officers contextualize the author’s transcript. Remember, important information like that should be included no matter which of these formats you use.
How to Brainstorm Content for Your Own COVID-19 Response:
Use this Values List to identify 3-5 values you gained (or strengthened) during the pandemic.
Brainstorm examples to demonstrate each value (e.g., to show the value of “health” the example might be “I built a makeshift gym and designed a workout schedule”).
Write one bullet point per value. Keep it succinct, as in the examples above.
Here’s a simple Google doc template where you can brainstorm the ideas above.
How to Write About Coronavirus Using the (650-Word) Additional Information Section
First, here’s a comprehensive guide that describes what students typically use this section for. Know that writing about coronavirus in this section is also totally fine.
Next, ask yourself, “Am I sure I can’t fit everything into the 250-word Coronavirus/COVID-19 section described above?” The reason I ask that is that it’s tough for me to imagine a scenario in which your pandemic experience(s) would not fit into the space above. I suppose if you created a project that was so large in scope that you have lots of details that wouldn’t fit into 250 words, but I imagine this will apply to fewer than 5% of students. But if that’s you, then by all means, use this section.
If you do use this section, here are some general tips:
When you’re ready to brainstorm content for that section, use the simple three-step process described above where it says, “How to Brainstorm Content for Your Own COVID-19 Response.”
Probably keep the tone straightforward and factual. Value content over poetry. This is, after all, the Additional Information section.
Probably don’t write a whole 650-word essay on your coronavirus experience. Why?
a) What can be communicated in a 650-word essay can probably be communicated in 250 words in the coronavirus-specific section (see above).
b) It’s likely to be a very common essay topic (more on that below), so writing a full-length essay may lead to blending in more than standing out.
c) Again, this is the additional information (and not the additional essay) section.
Note that I say probably in the bullet points above. Could there be an exception to these tips? Something I’m not thinking about? Absolutely. If you are that exception, rock on. (In fact, email me and let me know if you feel you’re the exception, and please share with me what you wrote: [email protected] )
Again, here’s some guidance on how to use the Additional Information section in general.
How to Write About Coronavirus in Your College Essay (i.e., Your 650-Word Personal Statement)
Quick recap in case you skipped straight to this section:
Probably use the coronavirus-specific 250-word section on the Common App. That may be enough space to say what you want to say. Read the section above to see if that might be true for you.
Whatever doesn’t fit there, you can probably fit into the Additional Info section.
If you’ve read both of the sections above and you’re still feeling like you want to write about your pandemic experience in your personal statement, ask yourself if you want to devote:
Part of your personal statement to your pandemic experience (maybe because it connects to a topic you were considering anyway) or
All of your personal statement to your pandemic experience (maybe because you’ve faced extraordinary challenges)
If the answer is part (because you don’t want to potentially be defined by your pandemic experience in your reader’s eyes), I’d recommend using the Montage Structure and devoting a paragraph of your essay to your pandemic experience.
In other words, think of it as a chapter in your life as opposed to the whole book.
If the answer is all (maybe because you’ve faced significant challenges), I’d recommend the Narrative Structure .
Here’s how to write both of these, beginning with ...
How to Use Montage Structure to Describe Your Pandemic Experience in Part of Your Essay
Before you start writing about the pandemic, I’d recommend first brainstorming a variety of topics that might show different values/skills/qualities that you’ll bring with you to a college campus. You can do that using the exercises on this page in the section called “My favorite resources for brainstorming everything you'll need for your college application.” Each exercise will take you 5-20 minutes but will set you up for your entire application. In fact, you may want to bookmark that link because a) I’ll refer to it a couple of times below, plus b) the resources on that page will probably answer a lot of other college application questions you’ll have.
Once you’ve found a great non-pandemic-related topic that captures some of the magic of who you are, ask yourself ...
What was my main take-away from the pandemic?
A simple way to figure that out is to look at this List of Needs and identify 1-2 main needs that became more apparent to you during the pandemic.
Example: Maybe you realized how much you needed community . Or structure . Or contribution .
Whatever need(s) you identify, next answer: How did I work to meet that need during the pandemic?
In other words: How did you meet your need for community? Or structure? Or contribution? (Or whatever value you’ve picked.) What did you actually do ?
Once you’ve identified that, answer: What did I learn? Or how did I grow?
A great way to figure out what you learned or how you grew is to pick from this List of Values .
Try to identify 1-3 values you connected with more deeply as a result of your work to meet your needs.
Example Brainstorm:
Needs: Community + contribution
How I tried to meet these needs: Hosted a virtual open mic with my class where my peers took turns reading and sharing their pandemic experiences
What I learned: Some of my friends are really creative (or) that vulnerability can create closeness even when we can’t be together in person (or) you get the idea ...
Again, here’s a simple Google doc template where you can brainstorm the ideas above.
A Quick Word of Advice on How to Stand Out If You’re Writing About Coronavirus
Once you’ve identified a few potential (ideally, uncommon!) values, ask yourself: How could I work this idea or these ideas into the topic I’ve already thought of?
Again, make this just one part of the larger story of your life.
First, as I mentioned above, COVID-19 is likely to be a common topic this year. And while that doesn’t mean that you shouldn’t write about it, I do think it’s going to be a lot harder to stand out with this topic. So, if possible, brainstorm other possible ideas using the resources above before you commit to this as your topic.
Second, check in with yourself: Are you choosing this because some part of you believes, or someone told you, that it’s “better” to write about a challenge for your college essay? BECAUSE IT’S NOT TRUE. :) You’ll find many examples of amazing essays written by students who do not discuss significant challenges. (To see some, click this link and scroll down to the “Personal Statement Examples” link.)
Having said all this, if you still feel that describing your pandemic experience is The Best Way to Show Who You Are, then I recommend this structure:
Challenge(s) I faced based on the pandemic + their impact on me
What I did about it/them
What I learned/How I grew from the experience
Important: Make sure that only the start of your essay describes the challenges and their impact on you, then most of your essay is devoted to describing what you did about it and what you learned from the experience. Why? Because your goal with the personal statement is to demonstrate skills, qualities, values, and interests. If you’re committing to COVID-19 as a topic, you’re basically saying that you feel this is the best way to show the many sides of who you are. Is that true? Is this your deepest story?
If you’re not sure, complete this Feelings and Needs exercise . You’ll find out in about 15 minutes.
If you’re certain if this is your deepest story, still do the Feelings and Needs exercise . It’ll help you create an outline that you can use to write your personal statement.
That’s what I’ve got.
Feel free to email to share examples of what you’re working on with [email protected] , as I’ll likely publish a follow-up once we get deeper into the fall.
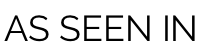
- Login / Sign Up
Support fearless, independent journalism
The election is here and the stakes are higher than ever. At Vox, we strive to bring clarity in uncertain times and help you understand what really matters. We know the impact of this election will be huge, and we believe you deserve to understand how the outcome will affect your life.
We rely on readers like you to fund our journalism. Will you support our work and become a Vox Member today?
Read these 12 moving essays about life during coronavirus
Artists, novelists, critics, and essayists are writing the first draft of history.
by Alissa Wilkinson

The world is grappling with an invisible, deadly enemy, trying to understand how to live with the threat posed by a virus . For some writers, the only way forward is to put pen to paper, trying to conceptualize and document what it feels like to continue living as countries are under lockdown and regular life seems to have ground to a halt.
So as the coronavirus pandemic has stretched around the world, it’s sparked a crop of diary entries and essays that describe how life has changed. Novelists, critics, artists, and journalists have put words to the feelings many are experiencing. The result is a first draft of how we’ll someday remember this time, filled with uncertainty and pain and fear as well as small moments of hope and humanity.
- The Vox guide to navigating the coronavirus crisis
At the New York Review of Books, Ali Bhutto writes that in Karachi, Pakistan, the government-imposed curfew due to the virus is “eerily reminiscent of past military clampdowns”:
Beneath the quiet calm lies a sense that society has been unhinged and that the usual rules no longer apply. Small groups of pedestrians look on from the shadows, like an audience watching a spectacle slowly unfolding. People pause on street corners and in the shade of trees, under the watchful gaze of the paramilitary forces and the police.
His essay concludes with the sobering note that “in the minds of many, Covid-19 is just another life-threatening hazard in a city that stumbles from one crisis to another.”
Writing from Chattanooga, novelist Jamie Quatro documents the mixed ways her neighbors have been responding to the threat, and the frustration of conflicting direction, or no direction at all, from local, state, and federal leaders:
Whiplash, trying to keep up with who’s ordering what. We’re already experiencing enough chaos without this back-and-forth. Why didn’t the federal government issue a nationwide shelter-in-place at the get-go, the way other countries did? What happens when one state’s shelter-in-place ends, while others continue? Do states still under quarantine close their borders? We are still one nation, not fifty individual countries. Right?
- A syllabus for the end of the world
Award-winning photojournalist Alessio Mamo, quarantined with his partner Marta in Sicily after she tested positive for the virus, accompanies his photographs in the Guardian of their confinement with a reflection on being confined :
The doctors asked me to take a second test, but again I tested negative. Perhaps I’m immune? The days dragged on in my apartment, in black and white, like my photos. Sometimes we tried to smile, imagining that I was asymptomatic, because I was the virus. Our smiles seemed to bring good news. My mother left hospital, but I won’t be able to see her for weeks. Marta started breathing well again, and so did I. I would have liked to photograph my country in the midst of this emergency, the battles that the doctors wage on the frontline, the hospitals pushed to their limits, Italy on its knees fighting an invisible enemy. That enemy, a day in March, knocked on my door instead.
In the New York Times Magazine, deputy editor Jessica Lustig writes with devastating clarity about her family’s life in Brooklyn while her husband battled the virus, weeks before most people began taking the threat seriously:
At the door of the clinic, we stand looking out at two older women chatting outside the doorway, oblivious. Do I wave them away? Call out that they should get far away, go home, wash their hands, stay inside? Instead we just stand there, awkwardly, until they move on. Only then do we step outside to begin the long three-block walk home. I point out the early magnolia, the forsythia. T says he is cold. The untrimmed hairs on his neck, under his beard, are white. The few people walking past us on the sidewalk don’t know that we are visitors from the future. A vision, a premonition, a walking visitation. This will be them: Either T, in the mask, or — if they’re lucky — me, tending to him.
Essayist Leslie Jamison writes in the New York Review of Books about being shut away alone in her New York City apartment with her 2-year-old daughter since she became sick:
The virus. Its sinewy, intimate name. What does it feel like in my body today? Shivering under blankets. A hot itch behind the eyes. Three sweatshirts in the middle of the day. My daughter trying to pull another blanket over my body with her tiny arms. An ache in the muscles that somehow makes it hard to lie still. This loss of taste has become a kind of sensory quarantine. It’s as if the quarantine keeps inching closer and closer to my insides. First I lost the touch of other bodies; then I lost the air; now I’ve lost the taste of bananas. Nothing about any of these losses is particularly unique. I’ve made a schedule so I won’t go insane with the toddler. Five days ago, I wrote Walk/Adventure! on it, next to a cut-out illustration of a tiger—as if we’d see tigers on our walks. It was good to keep possibility alive.
At Literary Hub, novelist Heidi Pitlor writes about the elastic nature of time during her family’s quarantine in Massachusetts:
During a shutdown, the things that mark our days—commuting to work, sending our kids to school, having a drink with friends—vanish and time takes on a flat, seamless quality. Without some self-imposed structure, it’s easy to feel a little untethered. A friend recently posted on Facebook: “For those who have lost track, today is Blursday the fortyteenth of Maprilay.” ... Giving shape to time is especially important now, when the future is so shapeless. We do not know whether the virus will continue to rage for weeks or months or, lord help us, on and off for years. We do not know when we will feel safe again. And so many of us, minus those who are gifted at compartmentalization or denial, remain largely captive to fear. We may stay this way if we do not create at least the illusion of movement in our lives, our long days spent with ourselves or partners or families.
- What day is it today?
Novelist Lauren Groff writes at the New York Review of Books about trying to escape the prison of her fears while sequestered at home in Gainesville, Florida:
Some people have imaginations sparked only by what they can see; I blame this blinkered empiricism for the parks overwhelmed with people, the bars, until a few nights ago, thickly thronged. My imagination is the opposite. I fear everything invisible to me. From the enclosure of my house, I am afraid of the suffering that isn’t present before me, the people running out of money and food or drowning in the fluid in their lungs, the deaths of health-care workers now growing ill while performing their duties. I fear the federal government, which the right wing has so—intentionally—weakened that not only is it insufficient to help its people, it is actively standing in help’s way. I fear we won’t sufficiently punish the right. I fear leaving the house and spreading the disease. I fear what this time of fear is doing to my children, their imaginations, and their souls.
At ArtForum , Berlin-based critic and writer Kristian Vistrup Madsen reflects on martinis, melancholia, and Finnish artist Jaakko Pallasvuo’s 2018 graphic novel Retreat , in which three young people exile themselves in the woods:
In melancholia, the shape of what is ending, and its temporality, is sprawling and incomprehensible. The ambivalence makes it hard to bear. The world of Retreat is rendered in lush pink and purple watercolors, which dissolve into wild and messy abstractions. In apocalypse, the divisions established in genesis bleed back out. My own Corona-retreat is similarly soft, color-field like, each day a blurred succession of quarantinis, YouTube–yoga, and televized press conferences. As restrictions mount, so does abstraction. For now, I’m still rooting for love to save the world.
At the Paris Review , Matt Levin writes about reading Virginia Woolf’s novel The Waves during quarantine:
A retreat, a quarantine, a sickness—they simultaneously distort and clarify, curtail and expand. It is an ideal state in which to read literature with a reputation for difficulty and inaccessibility, those hermetic books shorn of the handholds of conventional plot or characterization or description. A novel like Virginia Woolf’s The Waves is perfect for the state of interiority induced by quarantine—a story of three men and three women, meeting after the death of a mutual friend, told entirely in the overlapping internal monologues of the six, interspersed only with sections of pure, achingly beautiful descriptions of the natural world, a day’s procession and recession of light and waves. The novel is, in my mind’s eye, a perfectly spherical object. It is translucent and shimmering and infinitely fragile, prone to shatter at the slightest disturbance. It is not a book that can be read in snatches on the subway—it demands total absorption. Though it revels in a stark emotional nakedness, the book remains aloof, remote in its own deep self-absorption.
- Vox is starting a book club. Come read with us!
In an essay for the Financial Times, novelist Arundhati Roy writes with anger about Indian Prime Minister Narendra Modi’s anemic response to the threat, but also offers a glimmer of hope for the future:
Historically, pandemics have forced humans to break with the past and imagine their world anew. This one is no different. It is a portal, a gateway between one world and the next. We can choose to walk through it, dragging the carcasses of our prejudice and hatred, our avarice, our data banks and dead ideas, our dead rivers and smoky skies behind us. Or we can walk through lightly, with little luggage, ready to imagine another world. And ready to fight for it.
From Boston, Nora Caplan-Bricker writes in The Point about the strange contraction of space under quarantine, in which a friend in Beirut is as close as the one around the corner in the same city:
It’s a nice illusion—nice to feel like we’re in it together, even if my real world has shrunk to one person, my husband, who sits with his laptop in the other room. It’s nice in the same way as reading those essays that reframe social distancing as solidarity. “We must begin to see the negative space as clearly as the positive, to know what we don’t do is also brilliant and full of love,” the poet Anne Boyer wrote on March 10th, the day that Massachusetts declared a state of emergency. If you squint, you could almost make sense of this quarantine as an effort to flatten, along with the curve, the distinctions we make between our bonds with others. Right now, I care for my neighbor in the same way I demonstrate love for my mother: in all instances, I stay away. And in moments this month, I have loved strangers with an intensity that is new to me. On March 14th, the Saturday night after the end of life as we knew it, I went out with my dog and found the street silent: no lines for restaurants, no children on bicycles, no couples strolling with little cups of ice cream. It had taken the combined will of thousands of people to deliver such a sudden and complete emptiness. I felt so grateful, and so bereft.
And on his own website, musician and artist David Byrne writes about rediscovering the value of working for collective good , saying that “what is happening now is an opportunity to learn how to change our behavior”:
In emergencies, citizens can suddenly cooperate and collaborate. Change can happen. We’re going to need to work together as the effects of climate change ramp up. In order for capitalism to survive in any form, we will have to be a little more socialist. Here is an opportunity for us to see things differently — to see that we really are all connected — and adjust our behavior accordingly. Are we willing to do this? Is this moment an opportunity to see how truly interdependent we all are? To live in a world that is different and better than the one we live in now? We might be too far down the road to test every asymptomatic person, but a change in our mindsets, in how we view our neighbors, could lay the groundwork for the collective action we’ll need to deal with other global crises. The time to see how connected we all are is now.
The portrait these writers paint of a world under quarantine is multifaceted. Our worlds have contracted to the confines of our homes, and yet in some ways we’re more connected than ever to one another. We feel fear and boredom, anger and gratitude, frustration and strange peace. Uncertainty drives us to find metaphors and images that will let us wrap our minds around what is happening.
Yet there’s no single “what” that is happening. Everyone is contending with the pandemic and its effects from different places and in different ways. Reading others’ experiences — even the most frightening ones — can help alleviate the loneliness and dread, a little, and remind us that what we’re going through is both unique and shared by all.
- Recommendations
Most Popular
- 5 mysteries that might determine the 2024 election
- The Supreme Court decides not to toss out thousands of Pennsylvania ballots
- Elon Musk assures voters that Trump’s victory would deliver “temporary hardship”
- 2024 election violence is already happening
- Take a mental break with the newest Vox crossword
Today, Explained
Understand the world with a daily explainer plus the most compelling stories of the day.
This is the title for the native ad
More in Culture

What social media shadow ban rumors tell us about election anxiety.

In the new Netflix documentary “Martha,” her relentless drive becomes her most endearing characteristic.

The latest attempt to find love in the pods was good for Netflix, but bad for everyone else involved.

How hip-hop’s Dionysus rose to power, decade after decade.

Let’s not forget Trump’s decades of documented sexual misconduct.

Red 3 has been banned for use in cosmetics and topical drugs since 1990. Why is it still in our food?
An official website of the United States government
Official websites use .gov A .gov website belongs to an official government organization in the United States.
Secure .gov websites use HTTPS A lock ( Lock Locked padlock icon ) or https:// means you've safely connected to the .gov website. Share sensitive information only on official, secure websites.
- Publications
- Account settings
- Advanced Search
- Journal List

The COVID-19 pandemic: a global health crisis
Casey a pollard, michael p morran, andrea l nestor-kalinoski.
- Author information
- Article notes
- Copyright and License information
Correspondence: A.L. Nestor-Kalinoski ( [email protected] ).
Corresponding author.
Received 2020 Jul 21; Revised 2020 Sep 22; Accepted 2020 Sep 28; Issue date 2020 Nov 1.
Licensed under Creative Commons Attribution CC-BY 4.0 . Published by the American Physiological Society.
The novel coronavirus SARS-CoV-2 was identified as the causative agent for a series of atypical respiratory diseases in the Hubei Province of Wuhan, China in December of 2019. The disease SARS-CoV-2, termed COVID-19, was officially declared a pandemic by the World Health Organization on March 11, 2020. SARS-CoV-2 contains a single-stranded, positive-sense RNA genome surrounded by an extracellular membrane containing a series of spike glycoproteins resembling a crown. COVID-19 infection results in diverse symptoms and morbidity depending on individual genetics, ethnicity, age, and geographic location. In severe cases, COVID-19 pathophysiology includes destruction of lung epithelial cells, thrombosis, hypercoagulation, and vascular leak leading to sepsis. These events lead to acute respiratory distress syndrome (ARDS) and subsequent pulmonary fibrosis in patients. COVID-19 risk factors include cardiovascular disease, hypertension, and diabetes, which are highly prevalent in the United States. This population has upregulation of the angiotensin converting enzyme-2 (ACE2) receptor, which is exploited by COVID-19 as the route of entry and infection. Viral envelope proteins bind to and degrade ACE2 receptors, thus preventing normal ACE2 function. COVID-19 infection causes imbalances in ACE2 and induces an inflammatory immune response, known as a cytokine storm, both of which amplify comorbidities within the host. Herein, we discuss the genetics, pathogenesis, and possible therapeutics of COVID-19 infection along with secondary complications associated with disease progression, including ARDS and pulmonary fibrosis. Understanding the mechanisms of COVID-19 infection will allow the development of vaccines or other novel therapeutic approaches to prevent transmission or reduce the severity of infection.
Keywords: ARDS, COVID-19, genetics, GTPases, pulmonary fibrosis
CORONAVIRUSES AND SARS-C o V-2 GENETICS
Coronaviruses are a well-studied group of viruses in the Coronaviridae family that are known for their ability to infect a variety of hosts due to their capacity to evolve in epidemiological situations, including crossing species barriers, mutagenesis, tissue tropism, and pathogenicity ( 10b , 14 , 83 ). Coronaviruses are round, enveloped virions roughly 80–220 nm in diameter that contain a single-stranded, positive-sense RNA genome of ∼26–32 kb surrounded by an extracellular membrane containing a casing of spike glycoproteins ( 32 , 80 ). The term corona in Latin translates to crown and was given to these viruses due to the presence of the spike casing that resembled a “crown-like structure” using electron microscopy ( 37 ).
Coronaviruses have been implicated in human disease as early as the late 1960s, where they were identified as the causative agents in respiratory illnesses that presented with mild symptoms associated with the common cold ( 32 ). Seven strains of human coronaviruses have been characterized, four of which are known to infect the upper respiratory tract and cause mild symptoms, while the three others are known for their severe disease-causing characteristics of the lower respiratory tract including the following: SARS-CoV (severe acute respiratory syndrome), MERS-CoV (Middle East respiratory syndrome), and SARS-CoV-2 (COVID-19) ( 42 ). Since the emergence of the COVID-19 pandemic, data-sharing initiatives have led to the much needed generation of SARS-CoV-2 data, including complete reference genomes in the National Center for Biotechnology Information database ( NC_045512 .2), which contains the 29,903 bp genomic sequence ( 83 ).
While it is known that the RNA polymerase of viruses lack proofreading capacity, the ensuing result is a high mutation rate with low replicative fidelity. In contrast, the coronaviruses possess an exonuclease proofreading capability that has resulted in the expansion and maintenance of one of the largest known viral genomes at ∼30 kb ( 17 , 60 ). The large viral genome of SARS-CoV-2 codes for four structural proteins including the envelope, membrane, nucleocapsid, and spike glycoprotein, which play a role in both molecular characterization and host cell entry ( 23 , 35 ). The SARS-CoV-2 genome also includes 16 nonstructural proteins and 9 accessory proteins required for replication and pathogenesis ( 23 , 35 , 60 ). While SARS-CoV-2 and SARS-CoV are 75–80% identical ( 3 , 89 ), SARS-CoV-2 displays the highest sequence similarities with BatCoV at 96.2% ( 11 ). Global sequence comparison of SARS-CoV-2 isolates have expanded the literature and information known for this virus in a short period of time. Initial analysis of roughly 100 genomes of SARS-CoV-2 identified two major subtypes, designated L and S, which vary due to the presence of two linked single nucleotide polymorphisms ( 71 ). Interestingly, the L subtype is a derivative of the S type and was identified in ∼70% of the genomes compared with the S type in the remaining 30% ( 71 ). Phylogenic tree analysis of the L type suggests that the differences are related to a significantly higher mutation rate, which, consequently, results in higher transmission and/or replication rates ( 71 ). Furthermore, the SARS-CoV-2 virus has geographically diverse strains that seemingly vary in severity, mortality rate, and treatment options that were characterized using phylogenetic network analysis of 160 SARS-CoV-2 genomes ( 18 ). Three distinct viral clusters (A, B, and C) were identified with derivative subgroups, with cluster A sharing the closest similarity to the BatCoV genome. Clusters A and C are found predominantly in the Americas and Europe, while cluster C is found across East Asia ( 18 ).
INDIVIDUAL GENETIC PREDISPOSITION/SUSCEPTIBILITY
Throughout the progression of the COVID-19 pandemic, it is clear that not all infected patients are created equal. The diversity in symptoms, morbidity, genetics, age, and geographic location all play distinct roles in viral transmission. Understanding the genetic implications underlying severe COVID-19 infection requires complex biochemical and immunological studies. Previously identified immune-related genetic variants known to be associated with susceptibility to SARS-CoV ( 61 , 85 ), including mannose-binding lectin, basigin (CD147), C-C motif chemokine ligand 2 (CCL2), interleukin-12 and human leukocyte antigen (HLA) genes, might show promise due to the shared homology of the two viral genomes ( 41 , 69 , 73 , 78 ). Utilizing our current understanding of viral entry and pathophysiology in relation to viral infection has prompted research focused on host genetic factors that may help to mitigate differences in viral replication and the innate and adaptive immune responses triggered during viral infection ( 75 ). While angiotensin-converting enzyme-2 (ACE2) receptor expression seems promising as a genetic element that could relate to immunity, no polymorphisms or mutations in ACE2 related to spike protein binding resistance have been reported in populations ( 8 ). Although rare, ACE2 variants have been identified that alter the interaction between host cells and SARS-CoV-2 causing reduced affinity of SARS-CoV-2 binding ( 66 ). Along this same line of reasoning, the gene encoding the transmembrane serine protease 2 (TMPRSS2) protease responsible for spike protein priming for viral entry has received much attention. Cell lines expressing high amounts of TMPRSS2 are highly susceptible to SARS-CoV-2 infection ( 43 ). In addition, it is known that TMPRSS2 has 2 isoforms 1 with and 1 without a 37 amino acid long cytoplasmic tail, which is thought to interact with viral spike proteins and promote viral spreading within the host ( 90 ).
Monoclonal antibodies against the spike protein of COVID-19 could play a pivotal role in blocking the virus attachment, fusion, and entry into host cells ( 67 , 72 ). Antibodies against the receptor-binding domain (RBD) of the spike protein or antibodies that bind to the ACE2 receptor have been discussed as potential therapeutics ( 67 , 72 ). Furthermore, recombinant RBD proteins have been shown to strongly bind to the ACE2 receptor in human and bat cells ( 67 ). There are also studies targeting glycocalyx loss as a therapeutic target of the spike protein. Importantly, blocking these initial steps in viral entry and replication could block the downstream cascade of COVID-19 pathophysiology. This would effectively decrease the morality rate of the current pandemic as it reduces the viral load in patients. Additionally, these antibodies could be potential candidates for COVID-19 antiviral and vaccine development ( 67 ). However, this therapeutic method would have very little impact on the case rate or the infectious propensity of the virus.
In addition, genetic alterations in immune response elements will be important in identifying possible gene candidates that could control host inflammatory responses that elicit the cytokine storm to help reduce secondary complications of infection by altering expression and activity of cytokines like IL-1, IL-6, interferons, and others ( 10 ). HLA is known to be one of the most polymorphic antigen systems in the body. In silico studies point out that all known HLA genotypes A, B, and C have affinity to bind SARS-CoV-2 peptides ( 50 ). Furthermore, predictive alleles have been found to have a binding capability that can infer susceptibility or possibly impart some T-cell-based immune response ( 50 ). Further studies have reviewed the genetic association of COVID-19 infection based on blood type ( 47 ) and sex, with the number of X chromosomes having an effect on susceptibility and progression of infection ( 20 ).
COVID-19 PATHOPHYSIOLOGY
The novel coronavirus SARS-CoV-2 was originally identified as the causative agent for a series of atypical respiratory diseases in the Hubei Province of Wuhan, China in December of 2019. The disease SARS-CoV-2, which will be termed COVID-19 from herein, was officially declared a pandemic by the World Health Organization (WHO) on March 11, 2020 ( 82b ). According to the WHO, there are 28,637,952 positive COVID-19 cases and 917,417 deaths worldwide as of September 14th, 2020 ( 82a ). As shown in Table 1 , the United States had 6,571,867 total cases resulting in 195,053 deaths, as of September 16th, 2020 according to the Centers of Disease Control and Prevention ( 10b ). Highly populated states like California, Texas, Florida, and New York have the highest total number of cases exceeding 400,000, while less populated rural states such as Vermont, Wyoming, and Maine have total case numbers below 5,000 ( 10b ). This reflects the predilection of the virus for more densely populated areas, allowing for higher rates of transmission in crowded areas compared with rural communities that are less densely populated. This can be seen in New York wherein the number of total deaths was 32,765 out numbering both California’s and Texas’s total deaths at 28,794 ( Table 1 ).
United States SARS-CoV-2 Statistics
Updated as of 09/09/2020 ( 82a ) and all others updated as of 09/16/2020 ( 10a – 10c ).
The epidemiology of COVID-19 to date has been found to have disproportionate impacts on populations depending on sex and ethnicity. Table 1 highlights the differences in total cases and mortality by ethnicity, sex, and age. For example, in the United States ∼51.7% of total COVID-19 cases are female and 48.3% are male ( 10a ). In contrast, 54% of the total deaths in the United States are male compared with 46% female ( 10a ). The most significant predictor of poor outcome and mortality associated with COVID-19 is age. The mortality data in Table 1 include available data in nine different age brackets spanning 0–85 yr and above. Most notably, patients 50 yr and above in the United States have the highest mortality rates accounting for >94% of the total deaths due to COVID-19 ( Table 1 ; 10b , 10c ). In contrast, individuals 18–29 yr old have the highest percentage of total cases at 23.3% but only have a mortality rate of ∼0.5% ( 10b , 10c ). Older adults have higher rates of chronic health conditions that have been associated with poorer COVID-19 outcomes including hypertension, diabetes, coronary artery disease, and chronic kidney disease ( 62 ). These conditions place adults over 60 yr old at the highest risk of developing a complicated COVID-19 infection and mortality compared with younger cohorts without these conditions ( 62 ). Many patients with these conditions also take daily medications that interfere with the renin-angiotensin-aldosterone system (RAAS) such as angiotensin-converting enzyme (ACE) inhibitors for hypertension. This system has been implicated in COVID-19 infection and the virus’s ability to attach to host cells, causing dysregulated host cell responses, which subsequently results in worse outcomes ( 20 , 25 , 66 ).
Patients with COVID-19 often present with an array of symptoms that are similar to influenza that can make it difficult to diagnose. An epidemiological study of the first 41 patients infected with COVID-19 in Wuhan, China found that fatigue, cough, and fever were the most commonly reported symptoms ( 28 , 31 ). As a result, the general symptoms of COVID-19 are challenging to diagnose without reliable testing. Positive COVID-19 classifications include the following: asymptomatic, mild, moderate, severe, and critical. Asymptomatic patients test positive and exhibit no clinical symptoms while mild cases present with acute symptoms of respiratory tract infection and digestive complications. Moderate patients experience pneumonia, without noticeable hypoxemia, with lesions on chest computerized tomography (CT) scan. Severe patients experience pneumonia with detectable hypoxemia and CT lesions while critical patients experience acute respiratory distress syndrome (ARDS) along with possible shock, encephalopathy, myocardial injury, coagulation dysfunction, heart failure, and acute kidney injury ( 86 ). In a study of 80 patients hospitalized for COVID-19, over 90% had detectable ground glass opacities present on CT scan ( 31 , 84 ). A correlation was also found with the degree of inflammation seen on chest CT and lymphopenia (low white blood cell count), days of symptoms, and fever ( 84 ). Although these symptoms are often informative in diagnosis, COVID-19 has an unpredictable clinical course. As a result, 13.8% of positive patients had severe cases that required an in-patient hospital stay, with 4.7% requiring intensive care unit hospitalization and 2.3% of cases resulting in death ( 31 ). Taken together, these factors make COVID-19 difficult to manage and hard for clinicians to diagnose and predict clinical outcomes. Furthermore, real-time generation of data using artificial intelligence is an absolute priority to combat the spread, diagnosis, treatment, and categorized susceptibility to COVID-19 ( 1 ).
Understanding the pathophysiology of COVID-19 is critical to improving patient outcomes and determining how we can overcome the current pandemic. A key component to the virus being able to enter host cells and replicate is the ACE2 receptor, which is highly expressed in alveolar epithelial cells of the lung as confirmed by RNA-seq ( 91 ). The viral glycoprotein spike casing found on the exterior of a virus particle is responsible for eliciting viral entry into susceptible host cells ( 27 ). The process of viral entry requires priming of the spike protein by host expressed TMPRSS2, which interacts with the spike protein and cleaves it into two functional subunits known as S1 and S2 ( 27 , 43 , 66 ). The S1 subunit directly interacts with the ACE2 receptor, leaving the S2 subunit to facilitate viral fusion with the host cell membrane ( Fig. 1 ; 25 , 27 , 41a ). Internalization and replication of virus subsequently cause degradation of membrane-bound ACE2 receptors ( 27 ), which in turn causes an increase in angiotensin II (ANG II) and the angiotensin type 1 receptor (AT 1 R) ( Fig. 1 ). Angiotensinogen is cleaved by renin to angiotensin I (ANG I). ANG I is cleaved via ACE to ANG II, wherein it can freely interact with AT 1 R and angiotensin type 2 receptor (AT 2 R). Excess ANG I and II are hydrolyzed by ACE2 to become the heptapeptides ANG-(1-9)/ANG-(1-7) ( Fig. 1 ). Reduced or bound ACE2 is unable to hydrolyze ANG I/II, which results in an inability of the counterbalancing effects of the Mas receptor (Mas-R) to protect against detrimental disease/immune complications. As a result of COVID-19 infection, decreases in ACE2 cause elevated activity in the ANG II/AT 1 R axis, resulting in an inflammatory immune response ( 76 ). This deficiency leads to many adverse outcomes for patients including interstitial fibrosis, myocardial hypertrophy, endothelial fibrosis, and increased inflammation ( 76 ). Additionally, thrombosis and hypercoagulation secondary to platelet activation after lung epithelial damage are seen in patients with severe infections ( 39 , 86 ). Further consequences of hypercoagulation include disseminated intravascular coagulation, pulmonary embolisms, cardiac complications, and an increased risk of death ( 39 , 70 ). Coagulation is induced as a protective physiological control in response to vascular leak but in turn elicits dangerous consequences in COVID-19 patients. Often the physiologic response mechanisms to vascular leak and permeability fail, which allows for enhanced viral invasion, thus amplifying the problem in host cells on two separate fronts ( 86 ).
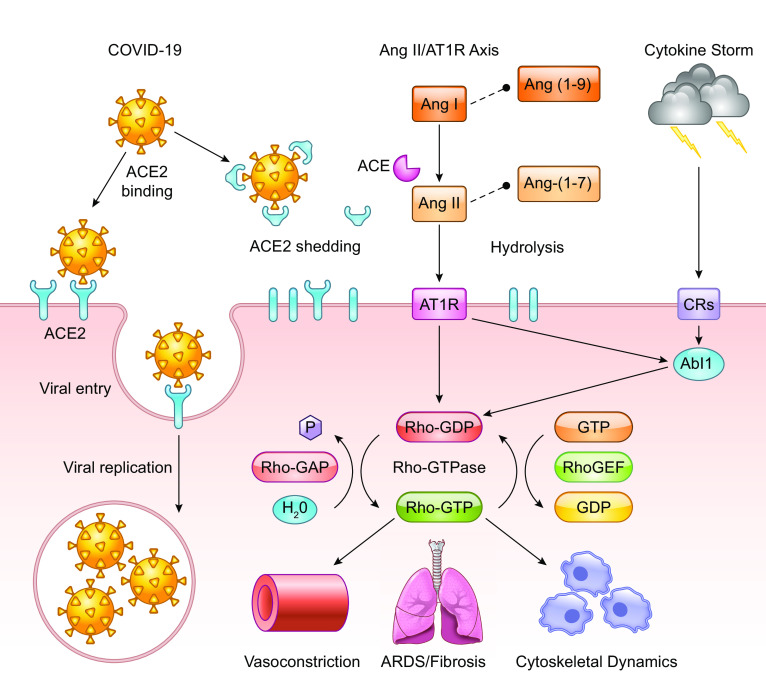
Biological effects of COVID-19 infection on angiotensin-converting enzyme 2 (ACE2) receptor and GTPase signaling pathways. The COVID-19 virus can bind and interact with both shed ACE2 and the cell membrane-bound ACE2 receptor. COVID-19 particles utilize and degrade membrane bound ACE2 receptors to gain entry into host cells. Virus particles also bind shed ACE2 causing a reduction in free ACE2 thus preventing the hydrolysis of ANG I/II into ANG-(1-9)/ANG-(1-7), which results in an imbalanced renin-angiotensin system that becomes skewed toward the ANG II/angiotensin type 1 receptor (AT 1 R) axis. COVID-19 produces an inflammatory response, i.e., the cytokine storm, which triggers cellular activation through cytokine receptors (CRs). Upon infection, these interactions favor detrimental complications such as acute respiratory distress syndrome (ARDS)/pulmonary fibrosis, vasoconstriction and alters cytoskeletal dynamics including cell proliferation, migration, and cytoskeletal composition. Intracellular elements such as Abelson murine leukemia viral oncogene homolog 1 kinase and Rho GTPase-associated proteins play a significant role in controlling polymerization of F-actin, maintaining the density of the extracellular matrix (ECM), and modulating myofibroblast proliferation, and the development of pulmonary fibrosis.
CARDIOVASCULAR DISEASE AND COVID-19
The highest risk factors for severe COVID-19 infection, including ARDS, is diabetes, hypertension, and a history of heart disease ( 76 ). Although the primary target of COVID-19 is the lungs, it can also have detrimental effects on the cardiovascular system. These comorbidities result in an upregulation of ACE2 on the cell surface of perivascular pericytes and cardiomyocytes, which is exploited by COVID-19 as the route of entry and infection ( 25 ). The leading cause of death in the United States is cardiovascular disease (CVD) causing more than 800,000 deaths in 2016 ( 20a ). A meta-analysis study in China found that COVID-19 causes acute cardiac injury in roughly 8.0% of patients, which poses concern for those that have a preexisting cardiac or metabolic condition ( 40 ). Cardiac injury may present as common arrhythmias, myocarditis, cardiogenic shock, and/or heart failure ( 24 , 49 ). Patients with prior cardiac history, including acute coronary syndrome and angina or myocardial infraction, have a higher risk for developing pneumonia and a decreased cardiac reserve that poses significant risks if they contract COVID-19 ( 40 , 88 ). The middle east respiratory syndrome coronavirus (MERS-CoV) is in the same corona virus family as COVID-19, has similar clinical outcomes, and has been extensively studied in patients with these comorbid conditions ( 5 ). In an analysis of 637 MERS-CoV patient cases, 30% had cardiac diseases and 50% had hypertension or diabetes ( 5 , 88 ). These cardiovascular disorders are highly prevalent in the United States, placing this vulnerable population in a higher risk category for acquiring severe infection with COVID-19. Patients with CVD may not have the ability to maintain cardiovascular function upon COVID-19 infection, leading to an increase in metabolic demand, exacerbating cardiovascular conditions thus increasing their risk for severe outcomes ( 68 ).
COVID-19 AND ACUTE RESPIRATORY DISTRESS SYNDROME
The host immune response to COVID-19 is similar to ARDS and therefore treatment modalities may be beneficial in treating COVID-19 patients. ARDS is defined clinically as bilateral neutrophilic infiltrates seen on imaging, acute hypoxia, and pulmonary edema ( 19 , 30 ). ARDS is caused by a dysregulated immune response with a fibroproliferative component due to excessive levels of cytokines, chemokines, and reactive oxygen species ( 30 ). ARDS-positive patients exhibit elevated levels of proinflammatory cytokines including IFN-y, IL-6, IL-12, and IL-1 compared with patients with uncomplicated COVID-19 infections ( 12 ). A study in ARDS positive mice confirmed these findings, wherein bronchoalveolar lavage fluid from ARDS positive mice strains had higher levels of TNF-α, IL-6, and vascular endothelial growth factor (VEGF) with reduced levels of IL-10 in comparison with controls ( 57 ). Similarly, patients hospitalized with severe COVID-19 infections have elevated cytokine profiles that are reflective of what defines a “cytokine storm.” The cytokine storm is a result of an uncontrolled immune response due to systemic inflammation and hemodynamic instability due to the abundance of proinflammatory cytokines that include IL-1, IL-6, IL-18, IFN-γ, and TNF-α ( Fig. 1 ) ( 65 ). As a result, new therapies are needed to thwart the immune response including nonconventional immunomodulation ( 22 ) to control the increase in proinflammatory cytokines that results in an accumulation of macrophages, neutrophils, and T cells from the circulation to the lung destroying the cell-cell interactions resulting in severe cases of ARDS. These findings suggest that patients suffering from ARDS and severe COVID-19 have a failed anti-inflammatory response that contributes to the excessive inflammatory damage caused by a host of proinflammatory cytokines wreaking havoc on lung tissue ( 58 ). Extensive damage to epithelial and endothelial cells of the lung triggers apoptotic destruction ( 12 ) leading to changes in the cellular junctions in alveolar tissue, thus increasing vascular permeability and ultimately alveolar fluid leak ( 30 ). Consequently, these cellular changes result in the pulmonary edema classically seen in ARDS patients ( 30 ), which is further complicated by an increase in dysregulated epithelial cell remodeling contributing to pulmonary fibrosis ( 12 ), a common cause of mortality in ARDS patients ( 30 ).
ABL1 AND VASCULAR PERMEABILITY
Abelson murine leukemia viral oncogene homolog 1 (Abl1) is a widely expressed nonreceptor tyrosine kinase that has been implicated in controlling cell morphology, growth, and survival ( 79 , 82 ). Abl1 is activated through a variety of receptor interactions and factors including cytokines, DNA damage, and oxidative stress ( 77 ). Abl1 plays a major role in modulating cytoskeletal dynamics influencing cell proliferation, cell survival, endocytosis, membrane trafficking, and cell-cell junctions and is also implicated in solid tumor proliferation and survival ( 34 ). Abl1 signals proteins that are critical to extracellular matrix (ECM) function and composition including the formation of actin stress fibers. These fibers interact with F-actin, inducing filopodia, which can alter cell-cell junctions ( 59 , 79 , 82 ).
Inhibition of Abl1 leads to increased Rho-Rock signaling, actomyosin contractility, and destabilization of cell-cell adhesions leading to an increase in barrier disruption ( 16 , 59 , 87 ). There is a direct implication of Abl1 as a therapeutic target to regulate GTPases in an effort to control ARDS and fibrosis as a result of disrupted endothelial barrier function and vascular leak in the lungs of ARDS patients ( 45 , 82 , 87 ). This critical association can be detrimental in ARDS, pulmonary fibrosis, and in severe cases of COVID-19 infection when vascular leak becomes uncontrolled and leads to sepsis ( 30 ). Multiple studies have investigated therapies to preserve endothelial barrier function. This includes the therapeutic use of low molecular weight heparin to combat the degradation of heparin sulfate by heparinase, thus protecting the endothelial barrier ( 7 ). Furthermore, the drug imatinib, an Abl1 inhibitor, has been investigated for possible repurposing and use for lung injury patients ( 36 , 82 ). One study found that pretreatment with imatinib protected against acute lung injury in mice ( 36 ) and may have potential to be repurposed in patients suffering from ARDS and/or COVID-19. Case studies report that imatinib resolved pneumonitis and pulmonary fibrosis secondary to antibiotics ( 9 , 59 ). Selective targeting of Abl1-based therapeutics needs further investigation to avoid potential negative side effects. For example, studies have shown that inhibiting Abl1 leads to increased endothelial permeability because of F-actin alternations and is amplified in cells undergoing cyclic stretch secondary to mechanical ventilation ( 38 , 59 ). As a result, increased vascular permeability will lead to an acceleration in vascular leak, exacerbating outcomes in ARDS patients.
PULMONARY FIBROSIS AND GTPase SIGNALING
While much is known about the progression of COVID-19 and ARDS, the mechanism of pathophysiology and associated treatment strategies are still under investigation. One such area includes GTPase signaling and its role in the development of ARDS and subsequent pulmonary fibrosis. Pulmonary fibrosis is caused by excessive fibroblasts and ECM protein deposits in the lungs, referred to as scarring of the lungs ( 4 ). Myofibroblasts are derived from resident fibroblasts and mesenchymal cells in the lung that express high amounts of smooth muscle actin ( 29 ) and are major players in the production of excess collagen leading to progressive fibrosis in patients ( 6 ). The overall ECM composition and stiffness have a direct impact on the degree of fibroblast migration, proliferation, and differentiation ( 4 ). Studies have shown that denser ECM substrates in later stages of disease show higher fibroblast migration levels compared with decreased fibroblasts migration in less stiff substrates as seen in earlier stages ( 6 ). One pathway with therapeutic implications in these physiological processes is the Rho GTPase signaling cascade ( 6 , 82 ).
Rho GTPase signaling has vast cellular implications in the control of actin and myosin stress fiber formation, regulation of cell adhesion molecules, cell migration, and common cellular functions ( 81 ). In addition, Rho GTPases play significant roles in cytoskeletal actin remodeling by polymerization and de-polymerization of monomeric G-actin leading to the conversion of F-actin ( 29 ). Increases in F-actin fibers causes stiffening of the ECM in patients suffering from ARDS leading to decreased vascular compliance ( 33 ). ARDS patients often require some form of oxygen supplementation due to severe hypoxemia. These measures often lead to hyperoxia and cause acute lung injury compounding damage to the lungs ( 30 , 44 ). Interestingly, hyperoxia in mice was found to activate the Rho/ROCK GTPase pathway and led to an increase in cell stiffness secondary to F-actin increase. However, when these mice were treated with Y-27632, a Rho inhibitor, the cytoskeletal changes in stiffness were prevented ( 81 ). These results suggest a possible connection in the control of GTPase signaling and ARDS and/or fibrosis complications seen in patients who require supplemental oxygen. Therefore, therapeutically modulating the increased activity of the GTPase cascade could decrease the adverse effects of ARDS pathogenesis secondary to ECM remodeling events.
As previously discussed, the Rho GTPase pathway regulates ECM density ( 81 , 82 ). This leads to the conclusion that higher activation levels of Rho and associated downstream targets lead to a higher levels of fibroblast proliferation. The ACE2 cascade is protective against lung fibrosis through activation of Rho GTPase pathways, while ACE is damaging and stimulates fibrosis in lung endothelial cells ( 46 ). These findings correlate to the virus’s predilection for patients with a history of obesity, hypertension, and CVD as these chronic conditions have been found to have lower levels of ACE2 at baseline ( 76 ). Therefore, the interplay between the ACE2/ACE and the Rho GTPase pathway may be an important association that could be a target for therapeutics to block lung fibrosis that results in ARDS and a majority of the mortality in COVID-19 patients. A study performed by Haung et al. proved this association by showing blockade of the Rho GTPase pathway inhibits matrix stiffness and alters stress fiber formation in fibroblasts ( 29 ). Therefore, Rho is actively involved in the underlying mechanism of pulmonary fibrosis by controlling proteins critical to modulating the ECM. A few significant trials have tested this theory for idiopathic pulmonary fibrosis by using nintedanib, a multikinase inhibitor, and pirfenidone, a small molecule antifibrotic, both of which were shown to reduce loss of lung functioning in pulmonary fibrosis patients ( 6 ).
STRATEGIES FOR SARS-C o V-2 THERAPEUTICS
Controlling the extensive spread and progression of SARS-CoV-2 has proven very difficult and will require a multidisciplinary approach with global collaboration. While certain areas of interest in SARS-CoV-2 remain unknown, past coronavirus knowledge provides scientists with the foundation for the development and/or repurposing of therapeutic interventions and vaccine development. Since the spike protein of each individual type of coronavirus is unique, this protein is currently being targeted in vaccine development as an approach to block initial entry of the virus ( 2 , 63 ). Multiple vaccines have entered clinical trials, the first of which is an RNA-based vaccine, mRNA-1273 ( 26 ). This vaccine entered phase I clinical trials on March 16, 2020 in collaboration with the National Institutes of Health (NIH), utilizing 45 healthy participants ranging in ages from 18 to 55 yr old ( 2 ). Although science has provided the foundational studies on vaccine development, the time needed to assess the safety and efficacy of vaccine candidates is a major bottleneck in the overall process.
While vaccines are being tested and manufactured, novel therapeutic treatments for the control and clinical management of COVID-19 infection are needed. Numerous approaches for treatment have been anecdotally reviewed in mainstream media; however, there are currently no Food and Drug Administration-approved medications for the treatment of COVID-19 infections ( 13 ). Still, there are a number of medications under evaluation for their effectiveness as potential antivirals that are recommended for use in the National Institutes of Health COVID-19 treatment guidelines ( 13 ). A noteworthy example of a current therapeutic intervention includes the use of convalescent plasma therapy ( 15 , 64 ). In this process, plasma-containing-neutralizing antibodies, removed from a donor who has previously recovered from a SARS-CoV-2 infection, are administered to infected patients to impart protection. Another unique therapeutic method involves treatment with soluble recombinant human ACE2 to disrupt viral entry via the spike protein-ACE2 interaction. Initial testing with recombinant ACE2 in simian cell lines and engineered human tissues shows promise in reducing viral load in a dose-dependent fashion ( 48 ). Finally, due to the high sense of urgency in clinical treatment of COVID-19 infection, the repurposing of known antiviral drugs has been explored with extreme caution, and the rationale are outlined in the NIH COVID-19 Treatment Guidelines ( 13 ). The treatment guidelines have the current recommendations either for or against the use of known antiviral drugs and the existing clinical trial data from the National Institutes of Health ( 13 ). Furthermore, implications for the use of some drugs have been identified using in silico databases that predict protein-protein interactions ( 23 , 74 ). Antiviral therapies contained in these studies include remdesivir, ivermectin, favipiravir, kaletra, and chloroquine/hydroxychloroquine with or without azithromycin ( 13 , 23 , 74 ).
The COVID-19 pandemic continues to pose a serious public health threat to nations around the world, as effective antiviral therapeutics or vaccines are yet to be developed. The primary goal in the COVID-19 pandemic is to limit transmission and define clinical management that improves the cure rate and effectively reduces the overall mortality rate. To achieve this goal, a complete understanding of all aspects of coronaviruses is needed to prevent or lessen their threat to society in the future. A thorough understanding of the epidemiology, pathophysiology and pandemic response efforts to combat COVID-19 is an invaluable lesson to society providing a protocol to fight future pandemics should they occur. Most importantly, scientific insights gained in the fight against COVID-19 will provide the evidence needed to develop vaccines and antiviral therapeutics that target viral entry, immune response and activation, and clinical management of secondary complications associated with severe infections.
M.P.M. and A.L.N-K. acknowledge funding support from the University of Toledo University Research Funding Opportunities (URFO) Program - Interdisciplinary Research Initiation Award I-127366-01.
DISCLOSURES
No conflicts of interest, financial or otherwise, are declared by the authors.
AUTHOR CONTRIBUTIONS
C.A.P., M.P.M., and A.L.N-K. prepared figures; C.A.P., M.P.M., and A.L.N-K. drafted manuscript; C.A.P., M.P.M., and A.L.N-K. edited and revised manuscript; C.A.P., M.P.M., and A.L.N-K. approved final version of manuscript.
- 1. Alimadadi A, Aryal S, Manandhar I, Munroe PB, Joe B, Cheng X. Artificial intelligence and machine learning to fight COVID-19. Physiol Genomics 52: 200–202, 2020. doi: 10.1152/physiolgenomics.00029.2020. [ DOI ] [ PMC free article ] [ PubMed ] [ Google Scholar ]
- 2. Amanat F, Krammer F. SARS-CoV-2 vaccines: status report. Immunity 52: 583–589, 2020. doi: 10.1016/j.immuni.2020.03.007. [ DOI ] [ PMC free article ] [ PubMed ] [ Google Scholar ]
- 3. Andersen KG, Rambaut A, Lipkin WI, Holmes EC, Garry RF. The proximal origin of SARS-CoV-2. Nat Med 26: 450–452, 2020. doi: 10.1038/s41591-020-0820-9. [ DOI ] [ PMC free article ] [ PubMed ] [ Google Scholar ]
- 4. Asano S, Ito S, Takahashi K, Furuya K, Kondo M, Sokabe M, Hasegawa Y. Matrix stiffness regulates migration of human lung fibroblasts. Physiol Rep 5: e13281, 2017. doi: 10.14814/phy2.13281. [ DOI ] [ PMC free article ] [ PubMed ] [ Google Scholar ]
- 5. Badawi A, Ryoo SG. Prevalence of comorbidities in the Middle East respiratory syndrome coronavirus (MERS-CoV): a systematic review and meta-analysis. Int J Infect Dis 49: 129–133, 2016. doi: 10.1016/j.ijid.2016.06.015. [ DOI ] [ PMC free article ] [ PubMed ] [ Google Scholar ]
- 6. Barkauskas CE, Noble PW. Cellular mechanisms of tissue fibrosis. 7. New insights into the cellular mechanisms of pulmonary fibrosis. Am J Physiol Cell Physiol 306: C987–C996, 2014. doi: 10.1152/ajpcell.00321.2013. [ DOI ] [ PMC free article ] [ PubMed ] [ Google Scholar ]
- 7. Buijsers B, Yanginlar C, Grondman I, de Nooijer A, Maciej-Hulme ML, Jonkman I, Janssen N, Rother N, de Graaf M, Pickkers P, Kox M, Joosten L, Nijenhuis T, Netea MG, Hillbrands L, van de Veerdonk F, Duivenvoorden R, de Mast Q, and van der Vlag J. Increased plasma heparanase activity in COVID-19 patients (Preprint). medRxiv; doi: 10.1101/2020.06.12.20129304. [ DOI ] [ PMC free article ] [ PubMed ]
- 8. Cao Y, Li L, Feng Z, Wan S, Huang P, Sun X, Wen F, Huang X, Ning G, Wang W. Comparative genetic analysis of the novel coronavirus (2019-nCoV/SARS-CoV-2) receptor ACE2 in different populations. Cell Discov 6: 11, 2020. doi: 10.1038/s41421-020-0147-1. [ DOI ] [ PMC free article ] [ PubMed ] [ Google Scholar ]
- 9. Carnevale-Schianca F, Gallo S, Rota-Scalabrini D, Sangiolo D, Fizzotti M, Caravelli D, Capaldi A, Anselmetti G, Palesandro E, D’Ambrosio L, Coha V, Obert R, Aglietta M, Grignani G. Complete resolution of life-threatening bleomycin-induced pneumonitis after treatment with imatinib mesylate in a patient with Hodgkin’s lymphoma: hope for severe chemotherapy-induced toxicity? J Clin Oncol 29: e691–e693, 2011. doi: 10.1200/JCO.2011.35.6733. [ DOI ] [ PubMed ] [ Google Scholar ]
- 10. Casanova JL, Su HC; COVID Human Genetic Effort . A global effort to define the human genetics of protective immunity to SARS-CoV-2 infection. Cell 181: 1194–1199, 2020. doi: 10.1016/j.cell.2020.05.016. [ DOI ] [ PMC free article ] [ PubMed ] [ Google Scholar ]
- 10a. Centers for Disease Control and Prevention . CDC COVID Data Tracker: Demographic Trends of COVID-19 cases and deaths in the US reported to CDC. https://covid.cdc.gov/covid-data-tracker/#demographics [16 September 2020].
- 10b. Centers For Disease Control and Prevention . Coronavirus Disease 2019 (COVID-19)- Cases, Data, & Surveillance. https://www.cdc.gov/coronavirus/2019-ncov/cases-updates/cases-in-us.html . [16 September 2020].
- 10c. Centers for Disease Control and Prevention . Provisional COVID-19 Death Counts by Sex, Age, and State. https://data.cdc.gov/NCHS/Provisional-COVID-19-Death-Counts-by-Sex-Age-and-S/9bhg-hcku [16 September 2020].
- 11. Ceraolo C, Giorgi FM. Genomic variance of the 2019-nCoV coronavirus. J Med Virol 92: 522–528, 2020. doi: 10.1002/jmv.25700. [ DOI ] [ PMC free article ] [ PubMed ] [ Google Scholar ]
- 12. Channappanavar R, Perlman S. Pathogenic human coronavirus infections: causes and consequences of cytokine storm and immunopathology. Semin Immunopathol 39: 529–539, 2017. doi: 10.1007/s00281-017-0629-x. [ DOI ] [ PMC free article ] [ PubMed ] [ Google Scholar ]
- 13. COVID-19 Treatment Guidelines Panel . Coronavirus Disease 2019 (COVID-19) Treatment Guidelines. National Institutes of Health. https://www.covid19treatmentguidelines.nih.gov/ [1 September 2020] [ PubMed ]
- 14. Decaro N, Mari V, Elia G, Addie DD, Camero M, Lucente MS, Martella V, Buonavoglia C. Recombinant canine coronaviruses in dogs, Europe. Emerg Infect Dis 16: 41–47, 2010. doi: 10.3201/eid1601.090726. [ DOI ] [ PMC free article ] [ PubMed ] [ Google Scholar ]
- 15. Duan K, Liu B, Li C, Zhang H, Yu T, Qu J, Zhou M, Chen L, Meng S, Hu Y, Peng C, Yuan M, Huang J, Wang Z, Yu J, Gao X, Wang D, Yu X, Li L, Zhang J, Wu X, Li B, Xu Y, Chen W, Peng Y, Hu Y, Lin L, Liu X, Huang S, Zhou Z, Zhang L, Wang Y, Zhang Z, Deng K, Xia Z, Gong Q, Zhang W, Zheng X, Liu Y, Yang H, Zhou D, Yu D, Hou J, Shi Z, Chen S, Chen Z, Zhang X, Yang X. Effectiveness of convalescent plasma therapy in severe COVID-19 patients. Proc Natl Acad Sci USA 117: 9490–9496, 2020. doi: 10.1073/pnas.2004168117. [ DOI ] [ PMC free article ] [ PubMed ] [ Google Scholar ]
- 16. Dudek SM, Garcia JG. Cytoskeletal regulation of pulmonary vascular permeability. J Appl Physiol (1985) 91: 1487–1500, 2001. doi: 10.1152/jappl.2001.91.4.1487. [ DOI ] [ PubMed ] [ Google Scholar ]
- 17. Ferron F, Subissi L, Silveira De Morais AT, Le NT, Sevajol M, Gluais L, Decroly E, Vonrhein C, Bricogne G, Canard B, Imbert I. Structural and molecular basis of mismatch correction and ribavirin excision from coronavirus RNA. Proc Natl Acad Sci USA 115: E162–E171, 2018. doi: 10.1073/pnas.1718806115. [ DOI ] [ PMC free article ] [ PubMed ] [ Google Scholar ]
- 18. Forster P, Forster L, Renfrew C, Forster M. Phylogenetic network analysis of SARS-CoV-2 genomes. Proc Natl Acad Sci USA 117: 9241–9243, 2020. doi: 10.1073/pnas.2004999117. [ DOI ] [ PMC free article ] [ PubMed ] [ Google Scholar ]
- 19. Fujishima S. Pathophysiology and biomarkers of acute respiratory distress syndrome. J Intensive Care 2: 32, 2014. doi: 10.1186/2052-0492-2-32. [ DOI ] [ PMC free article ] [ PubMed ] [ Google Scholar ]
- 20. Gemmati D, Bramanti B, Serino ML, Secchiero P, Zauli G, Tisato V. COVID-19 and Individual genetic susceptibility/receptivity: role of ACE1/ACE2 Genes, immunity, inflammation and coagulation. might the double X-chromosome in females be protective against SARS-CoV-2 compared to the single X-chromosome in males? Int J Mol Sci 21: 3474, 2020. doi: 10.3390/ijms21103474. [ DOI ] [ PMC free article ] [ PubMed ] [ Google Scholar ]
- 20a. Global Burden of Cardiovascular Diseases Collaboration; Roth GA, Johnson CO, Abate KH, Abd-Allah F, Ahmed M, Alam K, Alam T, Alvis-Guzman N, Ansari H, Ärnlöv J, Atey TM, Awasthi A, Awoke T, Barac A, Bärnighausen T, Bedi N, Bennett D, Bensenor I, Biadgilign S, Castañeda-Orjuela C, Catalá-López F, Davletov K, Dharmaratne S, Ding EL, Dubey M, Faraon EJ, Farid T, Farvid MS, Feigin V, Fernandes J, Frostad J, Gebru A, Geleijnse JM, Gona PN, Griswold M, Hailu GB, Hankey GJ, Hassen HY, Havmoeller R, Hay S, Heckbert SR, Irvine CM, James SL, Jara D, Kasaeian A, Khan AR, Khera S, Khoja AT, Khubchandani J, Kim D, Kolte D, Lal D, Larsson A, Linn S, Lotufo PA, Magdy Abd El Razek H, Mazidi M, Meier T, Mendoza W, Mensah GA, Meretoja A, Mezgebe HB, Mirrakhimov E, Mohammed S, Moran AE, Nguyen G, Nguyen M, Ong KL, Owolabi M, Pletcher M, Pourmalek F, Purcell CA, Qorbani M, Rahman M, Rai RK, Ram U, Reitsma MB, Renzaho AM, Rios-Blancas MJ, Safiri S, Salomon JA, Sartorius B, Sepanlou SG, Shaikh MA, Silva D, Stranges S, Tabarés-Seisdedos R, Tadele Atnafu N, Thakur JS, Topor-Madry R, Truelsen T, Tuzcu EM, Tyrovolas S, Ukwaja KN, Vasankari T, Vlassov V, Vollset SE, Wakayo T, Weintraub R, Wolfe C, Workicho A, Xu G, Yadgir S, Yano Y, Yip P, Yonemoto N, Younis M, Yu C, Zaidi Z, Zaki ME, Zipkin B, Afshin A, Gakidou E, Lim SS, Mokdad AH, Naghavi M, Vos T, Murray CJ;. The burden of cardiovascular diseases among US states, 1990-2016. JAMA Cardiol 3: 375–389, 2018. doi: 10.1001/jamacardio.2018.0385. [ DOI ] [ PMC free article ] [ PubMed ] [ Google Scholar ]
- 22. Golonka RM, Saha P, Yeoh BS, Chattopadhyay S, Gewirtz AT, Joe B, Vijay-Kumar M. Harnessing innate immunity to eliminate SARS-CoV-2 and ameliorate COVID-19 disease. Physiol Genomics 52: 217–221, 2020. doi: 10.1152/physiolgenomics.00033.2020. [ DOI ] [ PMC free article ] [ PubMed ] [ Google Scholar ]
- 23. Gordon DE, Jang GM, Bouhaddou M, Xu J, Obernier K, White KM, O’Meara MJ, Rezelj VV, Guo JZ, Swaney DL, Tummino TA, Hüttenhain R, Kaake RM, Richards AL, Tutuncuoglu B, Foussard H, Batra J, Haas K, Modak M, Kim M, Haas P, Polacco BJ, Braberg H, Fabius JM, Eckhardt M, Soucheray M, Bennett MJ, Cakir M, McGregor MJ, Li Q, Meyer B, Roesch F, Vallet T, Mac Kain A, Miorin L, Moreno E, Naing ZZ, Zhou Y, Peng S, Shi Y, Zhang Z, Shen W, Kirby IT, Melnyk JE, Chorba JS, Lou K, Dai SA, Barrio-Hernandez I, Memon D, Hernandez-Armenta C, Lyu J, Mathy CJ, Perica T, Pilla KB, Ganesan SJ, Saltzberg DJ, Rakesh R, Liu X, Rosenthal SB, Calviello L, Venkataramanan S, Liboy-Lugo J, Lin Y, Huang XP, Liu Y, Wankowicz SA, Bohn M, Safari M, Ugur FS, Koh C, Savar NS, Tran QD, Shengjuler D, Fletcher SJ, O’Neal MC, Cai Y, Chang JC, Broadhurst DJ, Klippsten S, Sharp PP, Wenzell NA, Kuzuoglu-Ozturk D, Wang HY, Trenker R, Young JM, Cavero DA, Hiatt J, Roth TL, Rathore U, Subramanian A, Noack J, Hubert M, Stroud RM, Frankel AD, Rosenberg OS, Verba KA, Agard DA, Ott M, Emerman M, Jura N, von Zastrow M, Verdin E, Ashworth A, Schwartz O, d’Enfert C, Mukherjee S, Jacobson M, Malik HS, Fujimori DG, Ideker T, Craik CS, Floor SN, Fraser JS, Gross JD, Sali A, Roth BL, Ruggero D, Taunton J, Kortemme T, Beltrao P, Vignuzzi M, García-Sastre A, Shokat KM, Shoichet BK, Krogan NJ. A SARS-CoV-2 protein interaction map reveals targets for drug repurposing. Nature 583: 459–468, 2020. doi: 10.1038/s41586-020-2286-9. [ DOI ] [ PMC free article ] [ PubMed ] [ Google Scholar ]
- 24. Gupta MD, Girish MP, Yadav G, Shankar A, Yadav R. Coronavirus disease 2019 and the cardiovascular system: Impacts and implications. Indian Heart J 72: 1–6, 2020. doi: 10.1016/j.ihj.2020.03.006. [ DOI ] [ PMC free article ] [ PubMed ] [ Google Scholar ]
- 25. Guzik TJ, Mohiddin SA, Dimarco A, Patel V, Savvatis K, Marelli-Berg FM, Madhur MS, Tomaszewski M, Maffia P, D’Acquisto F, Nicklin SA, Marian AJ, Nosalski R, Murray EC, Guzik B, Berry C, Touyz RM, Kreutz R, Wang DW, Bhella D, Sagliocco O, Crea F, Thomson EC, McInnes IB. COVID-19 and the cardiovascular system: implications for risk assessment, diagnosis, and treatment options. Cardiovasc Res 116: 1666–1687, 2020. doi: 10.1093/cvr/cvaa106. [ DOI ] [ PMC free article ] [ PubMed ] [ Google Scholar ]
- 26. Hodgson J. The pandemic pipeline. Nat Biotechnol 38: 523–532, 2020. doi: 10.1038/d41587-020-00005-z. [ DOI ] [ PubMed ] [ Google Scholar ]
- 27. Hoffmann M, Kleine-Weber H, Schroeder S, Krüger N, Herrler T, Erichsen S, Schiergens TS, Herrler G, Wu NH, Nitsche A, Müller MA, Drosten C, Pöhlmann S. SARS-CoV-2 cell entry depends on ACE2 and TMPRSS2 and is blocked by a clinically proven protease inhibitor. Cell 181: 271–280.e8, 2020. doi: 10.1016/j.cell.2020.02.052. [ DOI ] [ PMC free article ] [ PubMed ] [ Google Scholar ]
- 28. Huang C, Wang Y, Li X, Ren L, Zhao J, Hu Y, Zhang L, Fan G, Xu J, Gu X, Cheng Z, Yu T, Xia J, Wei Y, Wu W, Xie X, Yin W, Li H, Liu M, Xiao Y, Gao H, Guo L, Xie J, Wang G, Jiang R, Gao Z, Jin Q, Wang J, Cao B. Clinical features of patients infected with 2019 novel coronavirus in Wuhan, China. Lancet 395: 497–506, 2020. doi: 10.1016/S0140-6736(20)30183-5. [ DOI ] [ PMC free article ] [ PubMed ] [ Google Scholar ]
- 29. Huang X, Yang N, Fiore VF, Barker TH, Sun Y, Morris SW, Ding Q, Thannickal VJ, Zhou Y. Matrix stiffness-induced myofibroblast differentiation is mediated by intrinsic mechanotransduction. Am J Respir Cell Mol Biol 47: 340–348, 2012. doi: 10.1165/rcmb.2012-0050OC. [ DOI ] [ PMC free article ] [ PubMed ] [ Google Scholar ]
- 30. Huppert LA, Matthay MA, Ware LB. Pathogenesis of acute respiratory distress syndrome. Semin Respir Crit Care Med 40: 31–39, 2019. doi: 10.1055/s-0039-1683996. [ DOI ] [ PMC free article ] [ PubMed ] [ Google Scholar ]
- 31. Jin Y, Yang H, Ji W, Wu W, Chen S, Zhang W, Duan G. Virology, epidemiology, pathogenesis, and control of COVID-19. Viruses 12: 372, 2020. doi: 10.3390/v12040372. [ DOI ] [ PMC free article ] [ PubMed ] [ Google Scholar ]
- 32. Kahn JS, McIntosh K. History and recent advances in coronavirus discovery. Pediatr Infect Dis J 24, Suppl: S223–S227, 2005. doi: 10.1097/01.inf.0000188166.17324.60. [ DOI ] [ PubMed ] [ Google Scholar ]
- 33. Karki P, Birukova AA. Substrate stiffness-dependent exacerbation of endothelial permeability and inflammation: mechanisms and potential implications in ALI and PH (2017 Grover Conference Series). Pulm Circ 8: 2045894018773044, 2018. doi: 10.1177/2045894018773044. [ DOI ] [ PMC free article ] [ PubMed ] [ Google Scholar ]
- 34. Khatri A, Wang J, Pendergast AM. Multifunctional Abl kinases in health and disease. J Cell Sci 129: 9–16, 2016. doi: 10.1242/jcs.175521. [ DOI ] [ PMC free article ] [ PubMed ] [ Google Scholar ]
- 35. Kim D, Lee JY, Yang JS, Kim JW, Kim VN, Chang H. The architecture of SARS-CoV-2 transcriptome. Cell 181: 914–921.e10, 2020. doi: 10.1016/j.cell.2020.04.011. [ DOI ] [ PMC free article ] [ PubMed ] [ Google Scholar ]
- 36. Kim IK, Rhee CK, Yeo CD, Kang HH, Lee DG, Lee SH, Kim JW. Effect of tyrosine kinase inhibitors, imatinib and nilotinib, in murine lipopolysaccharide-induced acute lung injury during neutropenia recovery. Crit Care 17: R114, 2013. doi: 10.1186/cc12786. [ DOI ] [ PMC free article ] [ PubMed ] [ Google Scholar ]
- 37. Lai MM, Cavanagh D. The molecular biology of coronaviruses. Adv Virus Res 48: 1–100, 1997. doi: 10.1016/S0065-3527(08)60286-9. [ DOI ] [ PMC free article ] [ PubMed ] [ Google Scholar ]
- 38. Letsiou E, Rizzo AN, Sammani S, Naureckas P, Jacobson JR, Garcia JG, Dudek SM. Differential and opposing effects of imatinib on LPS- and ventilator-induced lung injury. Am J Physiol Lung Cell Mol Physiol 308: L259–L269, 2015. doi: 10.1152/ajplung.00323.2014. [ DOI ] [ PMC free article ] [ PubMed ] [ Google Scholar ]
- 39. Levi M, Thachil J, Iba T, Levy JH. Coagulation abnormalities and thrombosis in patients with COVID-19. Lancet Haematol 7: e438–e440, 2020. doi: 10.1016/S2352-3026(20)30145-9. [ DOI ] [ PMC free article ] [ PubMed ] [ Google Scholar ]
- 40. Li B, Yang J, Zhao F, Zhi L, Wang X, Liu L, Bi Z, Zhao Y. Prevalence and impact of cardiovascular metabolic diseases on COVID-19 in China. Clin Res Cardiol 109: 531–538, 2020. doi: 10.1007/s00392-020-01626-9. [ DOI ] [ PMC free article ] [ PubMed ] [ Google Scholar ]
- 41. Lin M, Tseng HK, Trejaut JA, Lee HL, Loo JH, Chu CC, Chen PJ, Su YW, Lim KH, Tsai ZU, Lin RY, Lin RS, Huang CH. Association of HLA class I with severe acute respiratory syndrome coronavirus infection. BMC Med Genet 4: 9, 2003. doi: 10.1186/1471-2350-4-9. [ DOI ] [ PMC free article ] [ PubMed ] [ Google Scholar ]
- 41a. Lippi G, Lavie CJ, Henry BM, Sanchis-Gomar F. Do genetic polymorphisms in angiotensin converting enzyme 2 (ACE2) gene play a role in coronavirus disease 2019 (COVID-19)? Clin Chem Lab Med 58: 1415–1422, 2020. doi: 10.1515/cclm-2020-0727. [ DOI ] [ PubMed ] [ Google Scholar ]
- 42. Lu G, Wang Q, Gao GF. Bat-to-human: spike features determining ‘host jump’ of coronaviruses SARS-CoV, MERS-CoV, and beyond. Trends Microbiol 23: 468–478, 2015. doi: 10.1016/j.tim.2015.06.003. [ DOI ] [ PMC free article ] [ PubMed ] [ Google Scholar ]
- 43. Matsuyama S, Nao N, Shirato K, Kawase M, Saito S, Takayama I, Nagata N, Sekizuka T, Katoh H, Kato F, Sakata M, Tahara M, Kutsuna S, Ohmagari N, Kuroda M, Suzuki T, Kageyama T, Takeda M. Enhanced isolation of SARS-CoV-2 by TMPRSS2-expressing cells. Proc Natl Acad Sci USA 117: 7001–7003, 2020. doi: 10.1073/pnas.2002589117. [ DOI ] [ PMC free article ] [ PubMed ] [ Google Scholar ]
- 44. Matthay MA, Zimmerman GA. Acute lung injury and the acute respiratory distress syndrome: four decades of inquiry into pathogenesis and rational management. Am J Respir Cell Mol Biol 33: 319–327, 2005. doi: 10.1165/rcmb.F305. [ DOI ] [ PMC free article ] [ PubMed ] [ Google Scholar ]
- 45. Mehta D, Malik AB. Signaling mechanisms regulating endothelial permeability. Physiol Rev 86: 279–367, 2006. doi: 10.1152/physrev.00012.2005. [ DOI ] [ PubMed ] [ Google Scholar ]
- 46. Meng Y, Li T, Zhou GS, Chen Y, Yu CH, Pang MX, Li W, Li Y, Zhang WY, Li X. The angiotensin-converting enzyme 2/angiotensin (1-7)/Mas axis protects against lung fibroblast migration and lung fibrosis by inhibiting the NOX4-derived ROS-mediated RhoA/Rho kinase pathway. Antioxid Redox Signal 22: 241–258, 2015. doi: 10.1089/ars.2013.5818. [ DOI ] [ PMC free article ] [ PubMed ] [ Google Scholar ]
- 47. Mohammadpour S, Torshizi Esfahani A, Halaji M, Lak M, Ranjbar R. An updated review of the association of host genetic factors with susceptibility and resistance to COVID-19. J Cell Physiol, 2020. doi: 10.1002/jcp.29868. [ DOI ] [ PMC free article ] [ PubMed ] [ Google Scholar ]
- 48. Monteil V, Kwon H, Prado P, Hagelkrüys A, Wimmer RA, Stahl M, Leopoldi A, Garreta E, Hurtado Del Pozo C, Prosper F, Romero JP, Wirnsberger G, Zhang H, Slutsky AS, Conder R, Montserrat N, Mirazimi A, Penninger JM. Inhibition of SARS-CoV-2 infections in engineered human tissues using clinical-grade soluble human ACE2. Cell 181: 905–913.e7, 2020. doi: 10.1016/j.cell.2020.04.004. [ DOI ] [ PMC free article ] [ PubMed ] [ Google Scholar ]
- 49. Murthy S, Gomersall CD, Fowler RA. Care for critically ill patients with COVID-19. JAMA 323: 1499–1500, 2020. doi: 10.1001/jama.2020.3633. [ DOI ] [ PubMed ] [ Google Scholar ]
- 50. Nguyen A, David JK, Maden SK, Wood MA, Weeder BR, Nellore A, and Thompson RF. Human leukocyte antigen susceptibility map for SARS-CoV-2 (Preprint). medRxiv; doi: 10.1101/2020.03.22.20040600. [ DOI ] [ PMC free article ] [ PubMed ]
- 57. Qin M, Qiu Z. Changes in TNF-α, IL-6, IL-10 and VEGF in rats with ARDS and the effects of dexamethasone. Exp Ther Med 17: 383–387, 2019. [ DOI ] [ PMC free article ] [ PubMed ] [ Google Scholar ]
- 58. Ragab D, Salah Eldin H, Taeimah M, Khattab R, Salem R. The COVID-19 cytokine storm; what we know so far. Front Immunol 11: 1446, 2020. doi: 10.3389/fimmu.2020.01446. [ DOI ] [ PMC free article ] [ PubMed ] [ Google Scholar ]
- 59. Rizzo AN, Aman J, van Nieuw Amerongen GP, Dudek SM. Targeting Abl kinases to regulate vascular leak during sepsis and acute respiratory distress syndrome. Arterioscler Thromb Vasc Biol 35: 1071–1079, 2015. doi: 10.1161/ATVBAHA.115.305085. [ DOI ] [ PMC free article ] [ PubMed ] [ Google Scholar ]
- 60. Robson F, Khan KS, Le TK, Paris C, Demirbag S, Barfuss P, Rocchi P, Ng WL. Coronavirus RNA proofreading: molecular basis and therapeutic targeting. Mol Cell 79: 710–727, 2020. doi: 10.1016/j.molcel.2020.07.027. [ DOI ] [ PMC free article ] [ PubMed ] [ Google Scholar ]
- 61. Saghazadeh A, Rezaei N. Immune-epidemiological parameters of the novel coronavirus - a perspective. Expert Rev Clin Immunol 16: 465–470, 2020. doi: 10.1080/1744666X.2020.1750954. [ DOI ] [ PMC free article ] [ PubMed ] [ Google Scholar ]
- 62. Shahid Z, Kalayanamitra R, McClafferty B, Kepko D, Ramgobin D, Patel R, Aggarwal CS, Vunnam R, Sahu N, Bhatt D, Jones K, Golamari R, Jain R. COVID-19 and older adults: what we know. J Am Geriatr Soc 68: 926–929, 2020. doi: 10.1111/jgs.16472. [ DOI ] [ PMC free article ] [ PubMed ] [ Google Scholar ]
- 63. Shang W, Yang Y, Rao Y, Rao X. The outbreak of SARS-CoV-2 pneumonia calls for viral vaccines. NPJ Vaccines 5: 18, 2020. doi: 10.1038/s41541-020-0170-0. [ DOI ] [ PMC free article ] [ PubMed ] [ Google Scholar ]
- 64. Shen C, Wang Z, Zhao F, Yang Y, Li J, Yuan J, Wang F, Li D, Yang M, Xing L, Wei J, Xiao H, Yang Y, Qu J, Qing L, Chen L, Xu Z, Peng L, Li Y, Zheng H, Chen F, Huang K, Jiang Y, Liu D, Zhang Z, Liu Y, Liu L. Treatment of 5 critically ill patients with COVID-19 with convalescent plasma. JAMA 323: 1582–1589, 2020. doi: 10.1001/jama.2020.4783. [ DOI ] [ PMC free article ] [ PubMed ] [ Google Scholar ]
- 65. Shimizu M. Clinical features of cytokine storm syndrome. In: Cytokine Storm Syndrome, edited by Cron RQ, Behrens EM. Cham, Switzerland: Springer International Publishing, 2019, p. 31–41. [ Google Scholar ]
- 66. Stawiski EW, Diwanji D, Suryamohan K, Gupta R, Fellouse FA, Sathirapongsasuti JF, Liu J, Jiang YP, Ratan A, Mis M, Santhosh D, Somasekar S, Mohan S, Phalke S, Kuriakose B, Antony A, Junutula JR, Schuster SC, Jura N, Seshagiri S. Human ACE2 receptor polymorphisms predict SARS-CoV-2 susceptibility (Preprint). bioRxiv; doi: 10.1101/2020.04.07.024752. [ DOI ] [ PMC free article ] [ PubMed ]
- 67. Tai W, He L, Zhang X, Pu J, Voronin D, Jiang S, Zhou Y, Du L. Characterization of the receptor-binding domain (RBD) of 2019 novel coronavirus: implication for development of RBD protein as a viral attachment inhibitor and vaccine. Cell Mol Immunol 17: 613–620, 2020. doi: 10.1038/s41423-020-0400-4. [ DOI ] [ PMC free article ] [ PubMed ] [ Google Scholar ]
- 68. Tan W, Aboulhosn J. The cardiovascular burden of coronavirus disease 2019 (COVID-19) with a focus on congenital heart disease. Int J Cardiol 309: 70–77, 2020. doi: 10.1016/j.ijcard.2020.03.063. [ DOI ] [ PMC free article ] [ PubMed ] [ Google Scholar ]
- 69. Tang F, Liu W, Zhang F, Xin ZT, Wei MT, Zhang PH, Yang H, Ly H, Cao WC. IL-12 RB1 genetic variants contribute to human susceptibility to severe acute respiratory syndrome infection among Chinese. PLoS One 3: e2183, 2008. doi: 10.1371/journal.pone.0002183. [ DOI ] [ PMC free article ] [ PubMed ] [ Google Scholar ]
- 70. Tang N, Li D, Wang X, Sun Z. Abnormal coagulation parameters are associated with poor prognosis in patients with novel coronavirus pneumonia. J Thromb Haemost 18: 844–847, 2020. doi: 10.1111/jth.14768. [ DOI ] [ PMC free article ] [ PubMed ] [ Google Scholar ]
- 71. Tang X, Wu C, Li X, Song Y, Yao X, Wu X, Duan Y, Zhang H, Wang Y, Qian Z, Cui J, Lu J. On the origin and continuing evolution of SARS-CoV-2. Natl Sci Rev 7: 1012–1023, 2020. doi: 10.1093/nsr/nwaa036. [ DOI ] [ PMC free article ] [ PubMed ] [ Google Scholar ]
- 72. Tian X, Li C, Huang A, Xia S, Lu S, Shi Z, Lu L, Jiang S, Yang Z, Wu Y, Ying T. Potent binding of 2019 novel coronavirus spike protein by a SARS coronavirus-specific human monoclonal antibody. Emerg Microbes Infect 9: 382–385, 2020. doi: 10.1080/22221751.2020.1729069. [ DOI ] [ PMC free article ] [ PubMed ] [ Google Scholar ]
- 73. Tu X, Chong WP, Zhai Y, Zhang H, Zhang F, Wang S, Liu W, Wei M, Siu NH, Yang H, Yang W, Cao W, Lau YL, He F, Zhou G. Functional polymorphisms of the CCL2 and MBL genes cumulatively increase susceptibility to severe acute respiratory syndrome coronavirus infection. J Infect 71: 101–109, 2015. doi: 10.1016/j.jinf.2015.03.006. [ DOI ] [ PMC free article ] [ PubMed ] [ Google Scholar ]
- 74. Uddin M, Mustafa F, Rizvi TA, Loney T, Al Suwaidi H, Al-Marzouqi AH, Kamal Eldin A, Alsabeeha N, Adrian TE, Stefanini C, Nowotny N, Alsheikh-Ali A, Senok AC. SARS-CoV-2/COVID-19: viral genomics, epidemiology, vaccines, and therapeutic interventions. Viruses 12: 526, 2020. doi: 10.3390/v12050526. [ DOI ] [ PMC free article ] [ PubMed ] [ Google Scholar ]
- 75. Vabret N, Britton GJ, Gruber C, Hegde S, Kim J, Kuksin M, Levantovsky R, Malle L, Moreira A, Park MD, Pia L, Risson E, Saffern M, Salomé B, Esai Selvan M, Spindler MP, Tan J, van der Heide V, Gregory JK, Alexandropoulos K, Bhardwaj N, Brown BD, Greenbaum B, Gümüş ZH, Homann D, Horowitz A, Kamphorst AO, Curotto de Lafaille MA, Mehandru S, Merad M, Samstein RM, Agrawal M, Aleynick M, Belabed M, Brown M, Casanova-Acebes M, Catalan J, Centa M, Charap A, Chan A, Chen ST, Chung J, Bozkus CC, Cody E, Cossarini F, Dalla E, Fernandez N, Grout J, Ruan DF, Hamon P, Humblin E, Jha D, Kodysh J, Leader A, Lin M, Lindblad K, Lozano-Ojalvo D, Lubitz G, Magen A, Mahmood Z, Martinez-Delgado G, Mateus-Tique J, Meritt E, Moon C, Noel J, O’Donnell T, Ota M, Plitt T, Pothula V, Redes J, Reyes Torres I, Roberto M, Sanchez-Paulete AR, Shang J, Schanoski AS, Suprun M, Tran M, Vaninov N, Wilk CM, Aguirre-Ghiso J, Bogunovic D, Cho J, Faith J, Grasset E, Heeger P, Kenigsberg E, Krammer F, Laserson U; Sinai Immunology Review Project . Immunology of COVID-19: current state of the science. Immunity 52: 910–941, 2020. doi: 10.1016/j.immuni.2020.05.002. [ DOI ] [ PMC free article ] [ PubMed ] [ Google Scholar ]
- 76. Verdecchia P, Cavallini C, Spanevello A, Angeli F. The pivotal link between ACE2 deficiency and SARS-CoV-2 infection. Eur J Intern Med 76: 14–20, 2020. doi: 10.1016/j.ejim.2020.04.037. [ DOI ] [ PMC free article ] [ PubMed ] [ Google Scholar ]
- 77. Wang JY. The capable ABL: what is its biological function? Mol Cell Biol 34: 1188–1197, 2014. doi: 10.1128/MCB.01454-13. [ DOI ] [ PMC free article ] [ PubMed ] [ Google Scholar ]
- 78. Wang K, Chen W, Zhou YS, Lian JQ, Zhang Z, Du P, Gong L, Zhang Y, Cui HY, Geng JJ, Wang B, Sun XX, Wang CF, Yang X, Lin P, Deng YQ, Wei D, Yang X-M, Zhu YM, Zhang K, Zheng ZH, Miao JL, Guo T, Shi Y, Zhang J, Fu L, Wang QY, Bian H, Zhu P, Chen ZN. SARS-CoV-2 invades host cells via a novel route: CD147-spike protein (Preprint). bioRxiv; doi: 10.1101/2020.03.14.988345. [ DOI ]
- 79. Wang L, Chiang ET, Simmons JT, Garcia JG, Dudek SM. FTY720-induced human pulmonary endothelial barrier enhancement is mediated by c-Abl. Eur Respir J 38: 78–88, 2011. doi: 10.1183/09031936.00047810. [ DOI ] [ PMC free article ] [ PubMed ] [ Google Scholar ]
- 80. Weiss SR, Navas-Martin S. Coronavirus pathogenesis and the emerging pathogen severe acute respiratory syndrome coronavirus. Microbiol Mol Biol Rev 69: 635–664, 2005. doi: 10.1128/MMBR.69.4.635-664.2005. [ DOI ] [ PMC free article ] [ PubMed ] [ Google Scholar ]
- 81. Wilhelm KR, Roan E, Ghosh MC, Parthasarathi K, Waters CM. Hyperoxia increases the elastic modulus of alveolar epithelial cells through Rho kinase. FEBS J 281: 957–969, 2014. doi: 10.1111/febs.12661. [ DOI ] [ PMC free article ] [ PubMed ] [ Google Scholar ]
- 82. Woodring PJ, Hunter T, Wang JY. Regulation of F-actin-dependent processes by the Abl family of tyrosine kinases. J Cell Sci 116: 2613–2626, 2003. doi: 10.1242/jcs.00622. [ DOI ] [ PubMed ] [ Google Scholar ]
- 82a. World Health Organization . Coronavirus disease (COVID-19) situation report-201. https://www.who.int/docs/default-source/coronaviruse/weekly-updates/wou-9-september-2020-cleared.pdf?sfvrsn=d39784f7_2 . [14 September 2020].
- 82b. World Health Organization . WHO timeline - COVID-19. https://www.who.int/news-room/detail/27-04-2020-who-timeline—covid-19 . [14 September 2020].
- 83. Wu F, Zhao S, Yu B, Chen YM, Wang W, Song ZG, Hu Y, Tao ZW, Tian JH, Pei YY, Yuan ML, Zhang YL, Dai FH, Liu Y, Wang QM, Zheng JJ, Xu L, Holmes EC, Zhang YZ. A new coronavirus associated with human respiratory disease in China. Nature 579: 265–269, 2020. doi: 10.1038/s41586-020-2008-3. [ DOI ] [ PMC free article ] [ PubMed ] [ Google Scholar ]
- 84. Wu J, Wu X, Zeng W, Guo D, Fang Z, Chen L, Huang H, Li C. Chest CT findings in patients with coronavirus disease 2019 and its relationship with clinical features. Invest Radiol 55: 257–261, 2020. doi: 10.1097/RLI.0000000000000670. [ DOI ] [ PMC free article ] [ PubMed ] [ Google Scholar ]
- 85. Yousefzadegan S, Rezaei N. Case report: death due to COVID-19 in three brothers. Am J Trop Med Hyg 102: 1203–1204, 2020. doi: 10.4269/ajtmh.20-0240. [ DOI ] [ PMC free article ] [ PubMed ] [ Google Scholar ]
- 86. Yuki K, Fujiogi M, Koutsogiannaki S. COVID-19 pathophysiology: a review. Clin Immunol 215: 108427, 2020. doi: 10.1016/j.clim.2020.108427. [ DOI ] [ PMC free article ] [ PubMed ] [ Google Scholar ]
- 87. Zandy NL, Playford M, Pendergast AM. Abl tyrosine kinases regulate cell-cell adhesion through Rho GTPases. Proc Natl Acad Sci USA 104: 17686–17691, 2007. doi: 10.1073/pnas.0703077104. [ DOI ] [ PMC free article ] [ PubMed ] [ Google Scholar ]
- 88. Zheng YY, Ma YT, Zhang JY, Xie X. COVID-19 and the cardiovascular system. Nat Rev Cardiol 17: 259–260, 2020. doi: 10.1038/s41569-020-0360-5. [ DOI ] [ PMC free article ] [ PubMed ] [ Google Scholar ]
- 89. Zhou Y, Hou Y, Shen J, Huang Y, Martin W, Cheng F. Network-based drug repurposing for novel coronavirus 2019-nCoV/SARS-CoV-2. Cell Discov 6: 14, 2020. doi: 10.1038/s41421-020-0153-3. [ DOI ] [ PMC free article ] [ PubMed ] [ Google Scholar ]
- 90. Zmora P, Moldenhauer AS, Hofmann-Winkler H, Pöhlmann S. TMPRSS2 isoform 1 activates respiratory viruses and is expressed in viral target cells. PLoS One 10: e0138380, 2015. doi: 10.1371/journal.pone.0138380. [ DOI ] [ PMC free article ] [ PubMed ] [ Google Scholar ]
- 91. Zou X, Chen K, Zou J, Han P, Hao J, Han Z. Single-cell RNA-seq data analysis on the receptor ACE2 expression reveals the potential risk of different human organs vulnerable to 2019-nCoV infection. Front Med 14: 185–192, 2020. doi: 10.1007/s11684-020-0754-0. [ DOI ] [ PMC free article ] [ PubMed ] [ Google Scholar ]
- View on publisher site
- Collections
Similar articles
Cited by other articles, links to ncbi databases.
- Download .nbib .nbib
- Format: AMA APA MLA NLM
Add to Collections
Thank you for visiting nature.com. You are using a browser version with limited support for CSS. To obtain the best experience, we recommend you use a more up to date browser (or turn off compatibility mode in Internet Explorer). In the meantime, to ensure continued support, we are displaying the site without styles and JavaScript.
- View all journals
- Explore content
- About the journal
- Publish with us
- Sign up for alerts
- Review Article
- Published: 17 October 2022
The effects of the COVID-19 pandemic on community respiratory virus activity
- Eric J. Chow ORCID: orcid.org/0000-0003-3349-9330 1 ,
- Timothy M. Uyeki ORCID: orcid.org/0000-0001-5338-8668 2 &
- Helen Y. Chu ORCID: orcid.org/0000-0001-8502-9600 1
Nature Reviews Microbiology volume 21 , pages 195–210 ( 2023 ) Cite this article
30k Accesses
279 Citations
632 Altmetric
Metrics details
- Epidemiology
- Infectious-disease diagnostics
- Viral infection
The emergence of severe acute respiratory syndrome coronavirus 2 (SARS-CoV-2) caused substantial global morbidity and deaths, leading governments to turn to non-pharmaceutical interventions to slow down the spread of infection and lessen the burden on health care systems. These policies have evolved over the course of the COVID-19 pandemic, including after the availability of COVID-19 vaccines, with regional and country-level differences in their ongoing use. The COVID-19 pandemic has been associated with changes in respiratory virus infections worldwide, which have differed between virus types. Reductions in respiratory virus infections, including by influenza virus and respiratory syncytial virus, were most notable at the onset of the COVID-19 pandemic and continued in varying degrees through subsequent waves of SARS-CoV-2 infections. The decreases in community infection burden have resulted in reduced hospitalizations and deaths associated with non-SARS-CoV-2 respiratory infections. Respiratory virus evolution relies on the maintaining of a diverse genetic pool, but evidence of genetic bottlenecking brought on by case reduction during the COVID-19 pandemic has resulted in reduced genetic diversity of some respiratory viruses, including influenza virus. By describing the differences in these changes between viral species across different geographies over the course of the COVID-19 pandemic, we may better understand the complex factors involved in community co-circulation of respiratory viruses.
Similar content being viewed by others
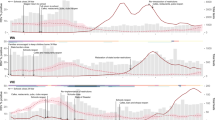
Off-season RSV epidemics in Australia after easing of COVID-19 restrictions
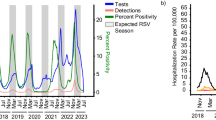
Deviations in RSV epidemiological patterns and population structures in the United States following the COVID-19 pandemic
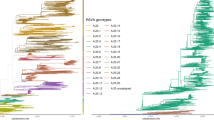
The genomic evolutionary dynamics and global circulation patterns of respiratory syncytial virus
Introduction.
The COVID-19 pandemic caused by severe acute respiratory syndrome coronavirus 2 (SARS-CoV-2) has upended the daily life of individuals around the world, with historic numbers of cases and deaths 1 . Early pandemic public health responses focused on non-pharmaceutical interventions (NPIs), actions that can be taken at the individual, environmental, community and country level to limit the spread of infection, given the significant health care burden of acute COVID-19 and the lack of COVID-19 vaccines or virus-specific therapeutics. NPIs have been the mainstay of pandemic influenza planning guidance issued by the World Health Organization (WHO) 2 , the Pan-American Health Organization 3 and the US Centers for Disease Control and Prevention 4 . The goal of near-simultaneous global implementation of NPIs (Table 1 ) during the COVID-19 pandemic was to slow down the community transmission of SARS-CoV-2, mitigate the burden of disease and the need for health care resources, and allow time to develop vaccines and treatments.
Since 2020, the COVID-19 pandemic and public health implementation of NPIs have had broad effects on individuals, communities and governments. Given that NPIs were designed to mitigate respiratory virus transmission, there have been notable disruptions to the typical seasonal circulation patterns of common respiratory virus infections, including by influenza virus and respiratory syncytial virus (RSV). The reduction in community respiratory virus activity, in turn, has led to other downstream effects, including an associated decreased prevalence of invasive Streptococcus pneumoniae infections (Box 1 ). These changes to the community prevalence of non-SARS-CoV-2 respiratory viruses are undoubtedly multifactorial, influenced in part by the collective implementation and ongoing use of NPIs, changes in health behaviours, reductions in travel, virus-specific transmission factors (Supplementary Table 1 ) and shifts in testing priorities and surveillance systems 5 , 6 . Virus–virus interactions, including viral interference, have been hypothesized as a factor explaining some of the changes in the community circulation of respiratory viruses 7 (Box 2 ). Public health responses have varied since the start of the pandemic as COVID-19 vaccinations became available and hospitalizations declined, resulting in a patchwork of community, regional and country-level differences in the ongoing use of NPIs, with corresponding effects on the circulation of respiratory viruses in the community. Documenting and understanding these changes in respiratory virus circulation during the COVID-19 pandemic can inform future public health responses.
In this Review, we summarize the epidemiology of common respiratory virus infections and then focus on the effect of SARS-CoV-2 and the use of NPIs in modifying the circulation, clinical burden and evolution of endemic respiratory virus infections.
Box 1 Changes in invasive pneumococcal disease during the COVID-19 pandemic
In addition to changes in viral pathogen activity, there have been reported global reductions in invasive pneumococcal disease (IPD) caused by Streptococcus pneumoniae , particularly among children younger than 5 years 149 , 150 , 151 , 152 , 153 , 154 , 155 , 156 . S. pneumoniae is a clinically important bacterial pathogen spread by respiratory droplets and can cause significant morbidity and death. Transmission can lead to colonization and a high carriage density 157 , which can in turn affect the risk of progression to invasive disease, including sepsis, meningitis and lower respiratory tract infections such as pneumonia 158 . Colonization of the respiratory tract by S. pneumoniae is influenced by vaccination 159 , 160 , 161 , host immunity 162 , respiratory microbiota interactions 163 and viral co-infections 157 , 163 . Specifically, influenza virus and other respiratory virus co-infections have been associated with increased S. pneumoniae carriage density through modifications of the microenvironment and facilitating S. pneumoniae growth in respiratory sites of colonization 157 , 163 . Furthermore, influenza virus co-infection has been associated with increased IPD risk 164 , 165 , 166 ; however, this synergistic relationship may differ across species of respiratory viruses that cause co-infection with S. pneumoniae 157 .
The decline in IPD during the pandemic is likely multifactorial, owing to factors such as reduction of person-to-person bacterial transmission through face mask mandates and social distancing 156 , reduction of bacterial burden among children from school closures 151 , 154 and changes in the circulation of viruses other than severe acute respiratory syndrome coronavirus 2 (SARS-CoV-2), which decreased the risk of co-infection 153 , 156 . The reduction of influenza virus infection and RSV infection cases mirrored reductions in IPD cases 153 . Although people with SARS-CoV-2-associated IPD experience severe disease outcomes 152 , S. pneumoniae and SARS-CoV-2 co-infections are uncommon 167 , 168 .
However, these reductions in IPD cases may not be sustained, as reports have shown increased incidence of IPD where non-pharmaceutical intervention measures were removed 169 . Furthermore, reduced paediatric vaccination rates during the COVID-19 pandemic 170 , including S. pneumoniae immunizations, may result in long-term increases in the community burden of IPD 171 .
Box 2 Viral interference
Respiratory viral infections can involve complex viral pathogen interactions when a host is infected with more than one type of respiratory virus or when a host is sequentially infected with different respiratory viruses. Among the different virus–virus interactions, viral interference is a direct or indirect antagonistic interaction between respiratory viruses that affects one virus’s ability to infect and cause disease in the host. Evidence of such inhibitory interactions was reported during the 2009 H1N1 influenza pandemic in Europe, during which preceding regional rhinovirus epidemics were temporally associated with unexplained and abrupt declines of influenza cases 172 , 173 . In turn, annual respiratory syncytial virus activity in France was delayed while infections due to pandemic influenza A(H1N1)pdm09 virus became widespread 174 . These early observations laid the foundation for subsequent studies exploring viral interference. Negative virus interactions have also been reported in other viral pairings 175 , 176 , and viral interference involving severe acute respiratory syndrome coronavirus 2 (SARS-CoV-2) is also thought to occur given the number of cases of SARS-CoV-2 but relatively few viral co-infections during the COVID-19 pandemic 177 .
Laboratory-based models have demonstrated plausible mechanisms by which viral interference could exist. For instance, infection with one virus stimulates a broad innate immune response and interferon release, which may inhibit subsequent respiratory viral infections 65 , 178 . Alternatively, simultaneous respiratory viral infections may lead to antagonistic effects; for example, viruses may compete for resources 179 , or the presence of one virus may drive the downregulation of cellular receptors that are necessary for viral entry in the host 180 . These effects can result in inhibition or delay of secondary infections, or the reduction of severe disease risk associated with subsequent infection.

Community circulation of non-SARS-CoV-2 respiratory viruses
The community co-circulation of respiratory viruses was common before the emergence of SARS-CoV-2. Established local, national and global surveillance systems, many of which measured medically attended respiratory virus infection cases, have helped monitor seasonal changes in circulation before the COVID-19 pandemic 8 , 9 , 10 . While influenza viruses and RSV have been prioritized for surveillance, the expanded use of multiplex molecular respiratory virus assays and the increased awareness of the public health burden of respiratory viruses have renewed interest in characterizing the epidemiology of respiratory viruses. Furthermore, the COVID-19 pandemic has highlighted the importance of community-based surveillance as a means to assess the burden of infection 11 , 12 , 13 .
Through the Seattle Flu Study (a community surveillance consortium in the Seattle, WA, USA, metropolitan area) community respiratory virus detection was monitored year-round 14 . With the community spread of SARS-CoV-2, there were associated circulation changes across the tested non-SARS-CoV-2 viral pathogens from March 2020 onwards, coinciding with community implementation of NPIs, including a stay-at-home ordinance implemented at the onset of the COVID-19 pandemic 15 , 16 (Fig. 1 ). Over time, the prevalence of SARS-CoV-2 and other respiratory viruses varied throughout the different phases of the COVID-19 pandemic, with changes in the detection of influenza virus, RSV, rhinovirus and respiratory enterovirus infections most commonly described. Characterizing these changes during the COVID-19 pandemic may help to shape public health recommendations to mitigate infection, especially in those most vulnerable to severe disease, and help our understanding of the interviral complexities that govern community co-circulation.
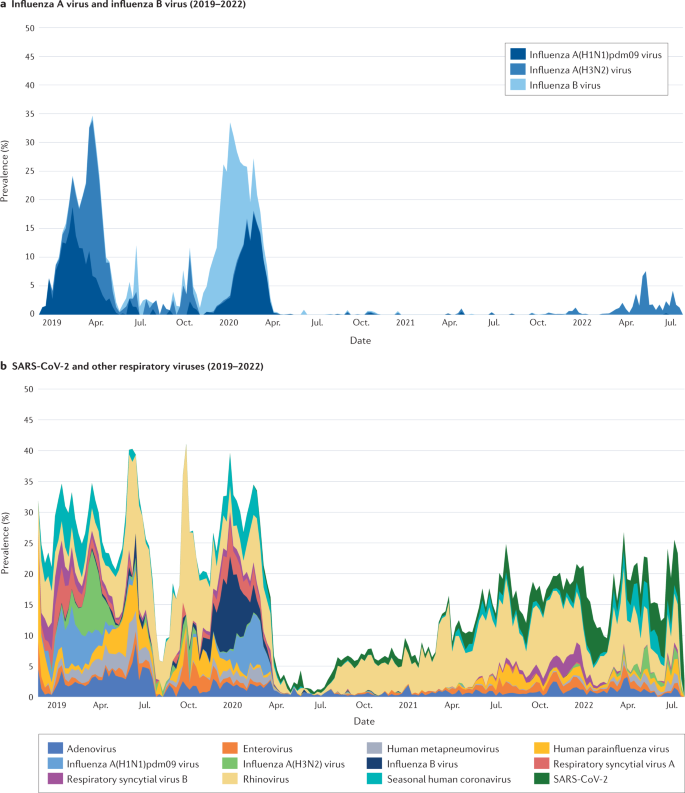
a | Prevalence of influenza A virus A and influenza B virus (2019–2022). b | Prevalence of severe acute respiratory syndrome coronavirus 2 (SARS-CoV-2) and other respiratory viruses. Included are respiratory specimens from 2018 epidemiological week 47 to 2022 epidemiological week 30; a total of 154,740 specimens were tested: 957 in 2018, 25,019 in 2019, 64,869 in 2020, 48,458 in 2021 and 15,437 in 2022. Respiratory specimens were tested with use of a custom-arrayed reverse transcription–PCR platform (Thermo Fisher) 16 . Adapted with permission from ref. 16 , Seattle Flu alliance.
Influenza virus circulation during the COVID-19 pandemic
Perhaps documented more than for any other respiratory virus, global influenza virus activity decreased markedly with the implementation of NPIs early in the COVID-19 pandemic 17 , 18 , 19 , 20 . Since 1952, the WHO has coordinated a global influenza surveillance system through the Global Influenza Surveillance and Response System, with 124 WHO member states 21 , 22 . The Centers for Disease Control and Prevention conducts seasonal influenza surveillance through syndromic surveillance for influenza-like illness (ILI) in laboratory, outpatient and hospital-based platforms, as well as through monitoring for deaths due to influenza and pneumonia in adult and paediatric populations 23 . The combined use of these and other systems around the globe has helped to clarify regional and temporal factors that affect the circulation of influenza virus, which are critical to influenza pandemic planning 4 .
Across different regions and hemispheres, there have been reduced cases of influenza since the start of the COVID-19 pandemic. While declines in the numbers of influenza cases may be due in part to changes in health care-seeking behaviours and influenza testing practices, specimen positivity rates have also shown an associated decline over this period 18 . Influenza vaccination coverage rates increased in adults and adolescents at the onset of the COVID-19 pandemic 24 while influenza vaccine uptake in those younger than 5 years decreased over the same period 24 . Influenza vaccination campaigns historically have focused on the youngest age groups 25 , 26 , 27 as children account for a substantial burden of influenza virus infections and are important contributors to influenza virus transmission in the community 25 , 26 , 27 , 28 , 29 . However, reductions in influenza virus activity reported by surveillance systems occurred despite evidence of decreased influenza vaccination coverage in children 24 , 30 .
Infections during the influenza season were reduced across a range of countries in the Northern Hemisphere. Influenza virus activity levels between October 2020 to early 2021 in the USA and Canada showed historically low levels, with US cases at their lowest since influenza surveillance data were first publicly available in 1997 (refs. 31 , 32 ). Similarly, in Mexico, where bimodal influenza seasons occur, national indicators of influenza virus activity showed a decline of influenza cases after broad NPI implementation began on 23 March 2020 (ref. 33 ). This included decreases in the frequency of ILI (see later), of clinical specimens that tested positive for influenza viruses, and of the numbers of severe influenza cases. In Bangladesh, the 2020–2021 influenza season was delayed and was shorter than prior seasons, with the start of the annual influenza epidemic occurring soon after NPIs were removed 34 . A modelling study of the timing of NPI implementation on influenza virus activity in China and the USA showed an associated decrease in influenza virus infections at the onset of the COVID-19 pandemic 19 , with influenza virus activity remaining suppressed in China throughout the remainder of 2020 (refs. 35 , 36 ). Surveillance reports in China from 2020–2021 show increased influenza virus activity relative to the previous year, with regional differences in the onset of peak activity; however, the degree of activity remained lower than in seasons before the COVID-19 pandemic 37 .
Similarly, data from the Southern Hemisphere showed much lower levels of influenza virus activity than in previous seasons during the months that typically mark the beginning of the influenza season 18 . Influenza surveillance sites in Australia, Chile and South Africa found that the influenza virus per cent positivity of clinical specimens was markedly lower from April to July 2020 compared with data from the 2017–2019 influenza seasons 18 . In Australia, this pattern of low influenza virus activity continued through to September 2020, despite variation in the implemented NPI strategies across the country 6 .
Global influenza virus activity remained low during the 2021–2022 influenza season, with low influenza virus numbers (as well as of most viruses) detected overall in northern temperate zone countries and countries in the tropics and subtropics 38 . However, there was a relative increase in influenza virus activity, particularly in temperate zone countries, compared with the 2020–2021 influenza season 38 . In China, influenza B virus predominated during the 2021–2022 influenza season, while other Asian countries reported cases of both influenza A virus and influenza B virus 38 .
How influenza virus activity will change over the remainder of the COVID-19 pandemic is unknown, especially with the variable lifting of travel restrictions, changes in NPI implementation policies, return to in-person learning, behavioural changes (such as variable compliance with masking), waning population immunity to influenza viruses, the emergence of new SARS-CoV-2 variants and subvariants, the evolving epidemiology of COVID-19 and challenges associated with influenza vaccine strain selection with low levels of circulating virus 39 .
Community circulation is thought to be driven in part by household transmission of influenza viruses 40 , with school-aged children playing a significant role in these infections 41 . However, despite the reopening of schools in some communities, early surveillance data during the 2021–2022 influenza season in the Northern Hemisphere indicated a return of only sporadic cases of influenza virus infections but not the same degree of activity observed before the COVID-19 pandemic in Canada 42 and the USA 8 . These examples show that personal-level NPIs, such as social distancing or face mask use, are associated with low influenza virus community circulation.
But even in communities where virus circulation is low, influenza outbreaks may still occur. An influenza A(H3N2) outbreak on a US university campus highlighted the effect of an abrupt return of community influenza virus circulation 43 . Data from the Seattle Flu Study identified a spike in influenza A(H3N2) virus prevalence in the spring of 2022, coinciding with the easing of pandemic NPIs 16 . This occurred at a time typically associated with the end of the traditional annual influenza season.
Moreover, several countries in the Southern Hemisphere reported influenza virus activity that was different from that in the pre-COVID-19 pandemic period. In Brazil, an unusual interseasonal influenza A(H3N2) epidemic occurred between October and November 2021 (ref. 44 ), while in Argentina influenza virus activity was increased in 2022, as reported in epidemiological week 17 (ref. 45 ). In June 2022, Australia experienced an early epidemic surge of seasonal influenza virus activity that peaked in May and June, with higher numbers of reported cases than during the three influenza seasons before the emergence of SARS-CoV-2 (ref. 46 ). It is unclear whether this is a reflection of the removal of COVID-19 pandemic NPIs or whether it was related to factors specific to that region.
RSV circulation during the COVID-19 pandemic
With COVID-19 NPIs in place, case counts decreased significantly across geographic regions and climate zones 31 , 32 , 47 , 48 , 49 , 50 . The effect of stay-at-home orders during the early pandemic decreased non-household social interactions, thereby reducing viral introductions and secondary household transmission. Because RSV is often transmitted through close contact in a childcare facility or school, the effect of these stay-at-home orders was likely to have had a more pronounced effect on the spread of RSV in paediatric populations, as well as onward transmission to groups at increased risk of infection, such as infants and adults aged 65 and older.
In Tokyo, where RSV outbreaks occur in summer and autumn months, no RSV outbreaks were observed in 2020 (ref. 51 ). However, in 2021, the Tokyo metropolitan area had the largest increase in RSV cases since RSV surveillance was established in 2003 (ref. 51 ). The rapid resurgence of RSV infections was thought to be due in part to the reduced population-level immunity as well as children’s re-engagement in social activities 51 .
Interseasonal RSV circulation has occurred during the COVID-19 pandemic, with uncharacteristic peaks during times of the year not typical of RSV seasonality 51 . In Australia and South Africa, where seasonal peaks generally occur during the winter, peaks of RSV cases were noted in the spring and summer of 2020, coinciding with the implementation and subsequent easing of community NPIs 48 , 52 , 53 . US cases of RSV infection decreased rapidly after NPI implementation and remained at historical low levels into early 2021 (ref. 54 ). However, RSV activity peaked in the USA during the spring and summer months of 2021, with variations by geographic region likely attributed to differences in implementation of community NPIs by states 55 .
Rhinovirus and respiratory enterovirus circulation during the COVID-19 pandemic
The case counts of rhinoviruses and respiratory enteroviruses decreased at the start of the pandemic. However, unlike for most of the other endemic respiratory viruses, the prevalence of rhinoviruses and respiratory enteroviruses quickly rebounded and persisted despite ongoing community NPI implementation.
Rhinoviruses and respiratory enteroviruses, which are non-enveloped viruses that are members of the same viral genus and are thus indistinguishable by most molecular tests, have been observed across multiple studies from different countries and regions. US national surveillance of respiratory viruses showed that rhinovirus and respiratory enterovirus detections decreased substantially between March and May 2020 but then returned to the seasonal levels of previous years 31 . In California, a sentinel surveillance system reported levels of rhinovirus and respiratory enterovirus activity that were similar to the levels in pre-COVID-19 pandemic seasons, with typical seasonal-level changes observed beginning in the autumn of 2021, after lower activity at the onset of the COVID-19 pandemic 56 . Persistence of non-enveloped respiratory viruses, including rhinovirus and respiratory enterovirus, was also seen in South Korea despite stringent social distancing practices during the pandemic 57 , 58 . The authors of these studies suggested that this was due to the non-enveloped virus structure’s resistance to environmental factors and prolonged shedding. Similarly, in a nationwide analysis of paediatric data in a Finnish infectious disease registry, rhinovirus and respiratory enterovirus detection initially decreased with early lockdown procedures and school closures but returned to typical levels as social distancing measures were eased 59 . During the winter season in Australia over the same period 6 , rhinovirus and respiratory enterovirus were noted to be present at above-average levels, and the levels increased as schools reopened 60 . Studies from tropical regions, including Brazil 61 and the Philippines 62 , where seasonality of respiratory viruses may differ from that in temperate regions, reported continued detection of rhinovirus and respiratory enterovirus among respiratory samples tested during the pandemic.
Collectively, these studies suggest that the inherent properties of rhinoviruses and respiratory enteroviruses, including the absence of a viral envelope, their stability on surfaces and the genomic diversity and number of strains that co-circulate, may allow infections to quickly increase and persist when the implementation of NPIs is relaxed. This is despite the continued suppression of other respiratory viruses by the remaining NPIs. A greater proportion of rhinovirus infections are minimally symptomatic or asymptomatic, compared with most other respiratory viruses, which may lead to ongoing transmission in settings where symptom screening was used as part of the criteria for school or work attendance. Given the sensitivity of rhinovirus and respiratory enterovirus to changes in implementation of NPIs, some have proposed using rhinovirus and respiratory enterovirus as a sentinel indicator of the stringency and effectiveness of NPI use 63 .
Interestingly, studies have identified an inverse relationship between levels of SARS-CoV-2 and rhinovirus and respiratory enterovirus in some locations. As rhinovirus and respiratory enterovirus cases returned to near pre-pandemic levels in both California 56 and South Korea 57 , their peaks and troughs were the inverse of those of SARS-CoV-2. While this finding may be incidental, viral interference 7 (Box 2 ) between the three viruses may be possible 64 . A similar virus–virus interaction has been reported at the host and population level between rhinovirus and influenza virus 65 .
Circulation of other non-SARS-CoV-2 respiratory viruses during the COVID-19 pandemic
Among other non-SARS-CoV-2 respiratory viruses, there has been variation in circulation changes by viral species during the COVID-19 pandemic.
Like influenza virus and RSV, seasonal human coronavirus 16 , 31 , 32 , 66 , 67 , human parainfluenza virus 16 , 32 , 60 , 67 , 68 and human metapneumovirus 16 , 32 , 60 , 67 , 68 experienced marked reductions in circulation at the onset of the COVID-19 pandemic when NPIs were in place. All three are negative-stranded RNA viruses in the family Paramyxoviridae , and share the features of having a viral envelope, circulating primarily in paediatric populations and having a clear seasonality. These viruses all rebounded as pandemic NPIs were lifted, thought to be due to school reopening and relaxation of NPIs leading to transmission 16 , 69 .
Adenoviruses and respiratory enteroviruses have both persisted despite NPIs. Although the levels of both decreased at the onset of the COVID-19 pandemic 16 , 32 , 67 , 70 , there were fewer fluctuations in case counts of these viruses compared with influenza virus and RSV despite shifting community policies in some localities 60 . In part, the persistence of adenovirus may be attributable to its lack of a viral envelope, and environmental stability, leading to persistent detection on surfaces, and extended shedding among infected individuals 71 . In Europe, relaxation of NPIs was associated with a rapid increase in enterovirus D68 cases between July and October 2021 (ref. 72 ).
Reports on human bocavirus early in the COVID-19 pandemic demonstrated different circulation patterns, with some reporting increasing positive testing rates 66 and others showing decreased frequencies 67 , 70 . Studies from China and South Korea found initial decreases in cases of human bocavirus 35 , 57 early in 2020, followed by a later resurgence beyond what was expected from previous season patterns in China 35 .
Data on human parechovirus circulation patterns during the COVID-19 pandemic are limited, with one study showing a decrease in human parechovirus detection in young children early in 2020 (ref. 67 ).
Health care burden of non-SARS-CoV-2 viruses
The health care burden of respiratory virus infections during the COVID-19 pandemic reflected the changes seen in respiratory virus community circulation.
From a health care utilization standpoint, the volume of emergency department (ED) visits and hospital admissions associated with non-SARS-CoV-2 respiratory virus infections decreased at the onset of the COVID-19 pandemic as broad NPIs were first implemented. One study estimated that community face mask use was associated with a significant decrease in ED visits for diseases associated with non-SARS-CoV-2 respiratory infections, including exacerbations of asthma and chronic obstructive pulmonary disease (COPD) 73 . As public health measures were lifted and some viruses returned, a corresponding increase in health care utilization was also seen.
While NPIs likely played an important part in medical visits, other factors may have also affected these measures of health care burden. Human behaviour and health care attendance changed during the COVID-19 pandemic, along with increases in telemedicine consultations, contributing to reports of fewer in-person clinic appointments 74 , 75 and ED visits 76 , 77 , 78 , 79 . Supply chain shortages and changes in respiratory virus testing priorities may have led to a prioritization of testing for SARS-CoV-2 in some localities until reagents became available, which may have been associated with less testing and therefore less detection of other respiratory viruses 20 . But even as patients returned for in-person care and health care systems increased their capacity to test for non-SARS-CoV-2 respiratory viruses, there were fewer ED visits and hospital admissions associated with non-SARS-CoV-2 respiratory viruses early in the COVID-19 pandemic.
ED visits associated with respiratory illnesses and non-SARS-CoV-2 viruses
The weekly number of US ED visits associated with respiratory illnesses and non-SARS-CoV-2 infections decreased early in the COVID-19 pandemic compared with the corresponding weeks of 2017–2019 (ref. 80 ). The number of ED visits associated primarily with respiratory concerns (including fever, cough and shortness of breath) initially increased between March and April 2020, but by the end of 2020, there were fewer non-SARS-CoV-2 respiratory ED visits than in previous years. The start of the COVID-19 pandemic was also associated with a decline in US paediatric ED visits compared with 2019, with the greatest decreases observed for non-SARS-CoV-2-associated respiratory illnesses 81 .
France 82 , Israel 83 , South Africa 84 , Turkey 85 and Finland 76 also reported decreases in ED visits associated with respiratory illness at the start of the COVID-19 pandemic. However, there was considerable variability in the number of ED visits for respiratory illnesses as the COVID-19 pandemic progressed, likely related to differences in country-specific implementation of NPIs.
Changes to ED visits associated with specific viral pathogens have also been noted, despite variations in testing patterns and implementation of COVID-19 NPIs. Sharp declines of both influenza virus-related ED visits 76 , 80 , 86 , 87 , 88 and RSV-related ED visits 76 , 80 , 88 were reported as NPIs were implemented, consistent with the decline in the community circulation of influenza virus and RSV. In addition, ED visits in the USA associated with other enveloped respiratory viruses, such as human metapneumovirus and human parainfluenza virus, showed a similar decline and remained low for the duration of 2020 (ref. 80 ). By contrast, the numbers of ED visits associated with the non-enveloped rhinovirus and respiratory enterovirus were similar and sometimes higher than what was observed in previous years, which is consistent with their persistence in the community 80 .
Hospital admissions associated with non-SARS-CoV-2 respiratory virus infections
Hospital admissions associated with non-SARS-CoV-2 respiratory virus infections showed declines similar to those of other measures of health care burden early in the COVID-19 pandemic. Hospitalization rates are an important public health measure of disease severity, and population-level hospital-based surveillance systems were established before the COVID-19 pandemic, particularly to monitor seasonal influenza virus activity.
As SARS-CoV-2 community spread began early in 2020, influenza-associated hospitalizations decreased internationally and continued to be at historically low levels until the 2021–2022 influenza season worldwide. Hospital-based surveillance early in the COVID-19 pandemic in Australia 6 , South Africa 48 and South Korea 58 showed reductions in influenza-associated hospitalizations throughout the remainder of 2020.
In the USA, where surveillance of influenza-associated hospitalization is conducted through the Influenza Hospitalization Surveillance Network (FluSurv-NET) 89 , the rates of influenza-associated hospitalization between February and March 2020 plateaued following community spread of SARS-CoV-2 (ref. 90 ). During the 2020–2021 influenza season, US surveillance sites reported a cumulative influenza-associated hospitalization rate of 0.8 per 100,000 population, the lowest rate of any season since 2005, when data collection began 91 . US hospitalization rates by age group in that year were not calculated given the low numbers of influenza-associated hospitalizations. Through the subsequent waves of SARS-CoV-2, there was regional variation in the ongoing use of COVID-19 NPIs, and by the 2021–2022 influenza season, influenza-associated hospitalization rates had returned to the levels seen in previous influenza seasons. As of 11 June 2022, the cumulative influenza-associated hospitalization rate in the US for all ages for the 2021–2022 influenza season was 17.1 per 100,000 population, compared with the pre-COVID-19 pandemic rate of 62.0 per 100,000 population during the 2016–2017 influenza season and 102.9 per 100,000 population during the 2019–2020 influenza season 8 .
Early in the COVID-19 pandemic, RSV-associated hospitalizations declined in countries in both the Northern Hemisphere 58 , 92 , 93 , 94 , 95 , 96 and the Southern Hemiphere 48 , 53 , 97 , where the typical RSV season was ending or just beginning, respectively. However, unlike influenza-associated hospitalizations, there has been variation in the trends of RSV-associated hospitalizations as COVID-19 NPIs were lifted. Many countries reported delayed returns of RSV-associated hospitalizations in comparison with pre-COVID-19 pandemic seasons, corresponding to community circulation patterns. In the USA, between October 2020 and April 2021, the Respiratory Syncytial Virus Hospitalization Surveillance Network 98 reported a lower RSV-associated hospitalization rate (0.3 per 100,000 persons) than in the two prior seasons (27.1 per 100,000 persons and 33.4 per 100,000 persons) 54 . Of the RSV-associated hospitalizations between October 2020 and May 2021, 77% were reported during April and May 2021, illustrating a shift in the typical peak of RSV-associated hospitalizations, which usually peak in the winter months 31 . One New York City area hospital reported a delay in its expected winter peak of RSV-associated hospitalizations during the 2020-2021 RSV season to the spring and summer months 99 . Potential reasons for this shift may be lower population-level immunity from lack of infections because of reduced circulation during the early days of the pandemic, leading to a larger susceptible pool of individuals who can be infected and transmit RSV.
Shifts in hospitalizations associated with the RSV season have also been seen in other countries, with variations in the degree of delay relative to previous seasons. In France, the 2020–2021 RSV season was characterized by a 3–4-month delay in the seasonal peak of RSV-associated hospitalizations 100 , 101 . Moreover, one Spanish hospital reported that RSV-associated bronchiolitis admissions were delayed until June 2021; in previous seasons, RSV-associated hospitalization peaks typically occurred between November and February 95 .
In the Southern Hemisphere, countries also experienced delays in RSV-associated hospitalizations during the 2020 winter months. Regional reports from Western Australia noted significant reductions in RSV-associated infections during the winter months 47 , 53 . However, an uncharacteristic interseasonal increase of RSV-associated ED visits and hospitalizations occurred in the spring and continued into the early summer months following the lifting of social distancing policies but before relaxation of restrictions on interstate travel 53 . In 2020, RSV-associated hospitalizations in Buenos Aires, Argentina, were very low during the winter months, when hospitalizations typically peak. In the winter season of 2021, a seasonal peak of RSV-associated hospitalizations returned following the reopening of schools in the community, but was delayed by 10 weeks compared with the 2016–2019 seasons 97 . The unpredictable onset and duration of the RSV season after initial COVID-19 pandemic NPIs were implemented complicated decisions around administration of palivizumab, a monoclonal antibody given to infants at increased risk of severe illness during the RSV season 102 .
In addition to the variation in RSV incidence shifts, there has been considerable geographic variation in the proportion of RSV infections requiring hospitalization, with some countries reporting higher rates and others lower rates as RSV re-emerged 100 . Moreover, one US study reported that children hospitalized with RSV during the 2020–2021 season required higher levels of care (81% versus 45%, respectively, admitted to an intensive care unit) and had a longer median length of hospitalization (4 days versus 3 days, respectively) than children hospitalized with RSV during the 2019–2020 season 99 .
Why these changes in the epidemiology of RSV occurred is not known, although these shifts may be in part due to waning population-level immunity. Infants are protected through transplacentally derived maternal antibody in the first several weeks of life. Low levels of RSV circulation will lead to waning population RSV antibody titres, and subsequently lead to lower levels of maternal antibody being transferred to infants during gestation 103 . This has the potential to result in a birth cohort with low levels of maternally derived antibody to experience their first RSV season, at a time when RSV circulation is much higher than in prior years. This would lead to both increased risk of infection and minimal neutralizing antibody titres to protect against severe disease. Simultaneously, there will also be older children who will also encounter their first RSV infection, and infections in young and older children could strain hospital capacity. Modelling studies have estimated that RSV hospitalizations in the post-pandemic period were twice as great as in a normal RSV season for children aged 1 year or younger, but to be highly dependent on NPIs in place and introductions of the virus from other regions 104 .
Data on hospital admissions due to other non-SARS-CoV-2 respiratory viruses are limited. Several reports demonstrate that rhinovirus-associated and respiratory enterovirus-associated hospitalizations in adults and children persisted or appeared to increase as the COVID-19 pandemic progressed, coinciding in part with the return of in-person school instruction for children 58 , 92 , 105 , 106 . A retrospective study of paediatric hospitalizations in one US children’s hospital reported a persistence of rhinovirus and respiratory enterovirus detection, while the number of other viruses decreased 92 . In a report on hospitalized adult patients from the UK, there was an initial decrease in rhinovirus-positive and respiratory enterovirus-positive test results between March and September 2020 (ref. 105 ). However, 2 weeks after the reopening of primary and secondary schools, there was a notable increase in the detection of rhinovirus and respiratory enterovirus in these hospitalized patients, reaching levels seen during the 2019 season 105 . In both China 106 and South Korea 58 , the numbers of rhinovirus-associated hospitalizations in the summer months of 2020 were higher than the numbers reported in previous seasons.
Whether increases in rhinovirus and respiratory enterovirus circulation reflect decreased interactions with other respiratory viruses, alteration of predominant rhinovirus species or serotypes, or an opportunistic change where rhinovirus infections are filling a niche left by decreases in the numbers of enveloped viruses is unknown. However, rhinovirus sequencing data early in the COVID-19 pandemic showed no significant changes in rhinovirus species predominance in comparison with previous seasons 57 , 106 .
Similar persistence patterns were also observed for other non-enveloped viruses, including adenovirus and human bocavirus, which demonstrated persistent but oscillating detections as the COVID-19 pandemic unfolded 58 .
Reports on hospitalizations associated with other non-SARS-CoV-2 respiratory viruses are limited, but some studies have shown similar declines at the start of the COVID-19 pandemic. In one US paediatric hospital, there was an abrupt decrease in non-SARS-CoV-2-associated hospitalizations after 21 April 2020 that continued through to December 2020 (ref. 92 ). In addition to no reported cases of influenza virus-associated or RSV-associated hospitalization, there were no hospitalizations for human metapneumovirus, human parainfluenza or seasonal human coronavirus infections through to 31 December 2020 (ref. 92 ). Hospital surveillance data from South Korea also demonstrated decreases in the monthly rate of detection of enveloped viruses, including human parainfluenza virus, seasonal human coronavirus and human metapneumovirus 58 .
Respiratory disease associated with non-SARS-CoV-2 viruses
The landscape of clinical respiratory disease burden not associated with SARS-CoV-2 infection has also changed during the COVID-19 pandemic. Respiratory syndromes and conditions such as otitis media, pneumonia and invasive bloodstream infections have been affected by shifts in viral pathogens, showing initial declines in frequency and alterations that match the clinical epidemiology of community-acquired respiratory viruses.
Acute respiratory illnesses and ILI
Declines in cases of acute respiratory illnesses (ARIs) and ILI were associated with the mitigation of non-SARS-CoV-2 respiratory viruses and have been reported most prominently among children. One multicentre ARI surveillance study in the USA showed a sharp decline in paediatric ARI cases in April 2020 after many communities had implemented stay-at-home orders 107 . This was largely due to the decline of influenza-associated and RSV-associated ARI.
In a multicentre prospective study of primary care paediatric practices in Germany between November 2020 and April 2021, rhinovirus was the most commonly detected respiratory virus among children with ARI, while SARS-CoV-2 and influenza virus infections were minimally detected 108 . Among children in Thailand seeking care, there was a notable increase in cases of ILI caused by rhinovirus in July 2020, which were replaced by RSV-associated ILI cases later in the season 109 . This suggests that although overall numbers of ILI cases declined, rhinovirus and RSV were important contributors to those cases that did require hospitalization.
All-cause outpatient ILI surveillance in the USA, conducted through ILI-Net, has shown general fluctuating patterns between the 2019–2020 and 2021–2022 influenza seasons 8 . The changes in the percentages of ILI outpatient visits are likely due in large part to the waves of SARS-CoV-2 infections in adults, but are possibly also due in part to non-SARS-CoV-2 respiratory viruses as endemic respiratory virus infections increased during 2021–2022 with the relaxation of NPIs. In a study of ILI in South Korea, the levels of respiratory virus causes fluctuated with community circulation, including initially lower levels of human bocavirus followed by increases in the detection rate towards the end of 2020 (ref. 57 ).
Community-acquired pneumonia incidence
Community-acquired pneumonia (CAP) causes substantial clinical burden and has both viral and bacterial aetiologies 110 , 111 . Many early COVID-19 pandemic reports showed a decrease in the incidence of non-COVID-19 CAP that was associated with the implementation of community NPIs 112 , 113 , 114 , 115 , 116 , 117 , 118 , 119 , 120 . Comparisons of these CAP cases early in the COVID-19 pandemic with CAP cases in periods during the pandemic but before NPI implementation and with CAP cases in previous seasons show a change in both bacterial and viral aetiologies 118 , 121 .
One multicentre study in Germany observed a seasonal decline in the frequencies of CAP cases associated with non-SARS-CoV-2 respiratory viruses, and these corresponded to a reduction in the diversity of the causative viral pathogen (that is, there were fewer types of respiratory viruses associated with CAP) 121 . For instance, from May 2020 through the remainder of the year, there was an abrupt reduction in the diversity of pathogens, with only rhinovirus-associated CAP as a persistent and lingering viral cause of non-COVID-19 CAP.
Bronchiolitis incidence
The burden and epidemiology of bronchiolitis, a common lower respiratory tract disease diagnosis in young children, has also been impacted by the effects of the COVID-19 pandemic.
While bronchiolitis can be associated with any respiratory virus infection, including SARS-CoV-2 infection and influenza virus infection, cases are commonly associated with RSV infection 122 . Many countries reported changes in the frequency of bronchiolitis cases that mirrored trends in community circulation of RSV during the COVID-19 pandemic. Bronchiolitis cases decreased in Northern Hemisphere countries, with low numbers of cases reported throughout much of the 2020–2021 respiratory virus season 94 , 95 , 96 , 120 , 123 , 124 , 125 , 126 .
The implementation of NPIs early in 2020 occurred at a time when seasonal cases of bronchiolitis were expected in the Southern Hemisphere. However, acute lower respiratory tract infections, including bronchiolitis, in children in Argentina remained low for the duration of the 2020 season, when bronchiolitis and other paediatric lower respiratory tract infection peaks were expected 127 . In Brazil, infant hospitalizations for respiratory disease, including bronchiolitis, declined during April and June 2020 (typically seasonal peaks occur from March to July) 128 . In Australia, fewer cases of hospitalized bronchiolitis and fewer bronchiolitis admissions to paediatric intensive care units were observed than in previous seasons 129 . However, sentinel surveillance of Australian hospital records when RSV epidemics reoccurred in late 2020 to early 2021 showed numbers similar to or higher than the numbers in previous seasons, confirming the mirrored incidence of bronchiolitis and RSV community transmission 130 .
Chronic respiratory disease incidence
Chronic respiratory diseases have also been impacted by the effects of the COVID-19 pandemic. Exacerbations of asthma and COPD are frequently associated with respiratory virus infections, and national lockdowns and pandemic NPIs were associated with fewer asthma hospital admissions in the early COVID-19 pandemic period 120 , 124 , 125 , 131 . In the UK, in addition to fewer asthma admissions, there were reductions in severe asthma exacerbations, with no significant changes in deaths due to asthma 132 . Frequencies of hospital admissions for exacerbations of COPD have also declined in South Korea and Slovenia 133 , 134 .
Several explanations have been proposed to explain these findings, including infections and non-infectious causes. Respiratory virus infections are frequently associated with exacerbations of chronic respiratory diseases. Therefore, the decreased community circulation, which is associated with behavioural changes and NPIs, may have led to fewer exacerbations attributed to respiratory viruses. One report noted that there was an increase in the number of prescriptions for inhaled and oral corticosteroid before lockdown was implemented, possibly due to concern about medication shortages or access during lockdown, and higher adherence to controller medication use 132 . Fewer hospitalizations due to asthma and COPD may also be due to improved home management of exacerbations. Other factors associated with fewer chronic respiratory disease exacerbations include additional changes in health-seeking behaviour, such as the use of telehealth visits that allowed management of exacerbations at home and reduction in air pollution from stay-at-home measures 135 .
Virus-associated severe illnesses and deaths
Severe illnesses associated with non-SARS-CoV-2 respiratory virus infections declined during some periods of the COVID-19 pandemic. For instance, only one influenza-associated paediatric death was reported in the USA during the 2020–2021 season, in contrast to144 deaths during the 2018–2019 season and 199 deaths during the 2019–2020 season. From October 2021 to April 2022, 24 influenza-associated paediatric deaths were reported in the USA, corresponding to increased influenza virus activity during the 2021–2022 season 8 .
Evolution of influenza viruses and other non-SARS-CoV-2 respiratory viruses
The global implementation of NPIs, broad community spread of SARS-CoV-2 and individual behaviour changes during the COVID-19 pandemic seem to have dramatically reduced the circulation of common respiratory viruses. However, many respiratory viruses returned as these measures were lifted, with the frequency of infections and circulation patterns influenced by regional differences in community NPIs. A hallmark of respiratory viruses is their ability to continue circulating despite the accumulated population immunity from infections, and in the case of influenza virus infections, the variable use of vaccines. Evolution is central to immune evasion by respiratory viruses, and is facilitated by genetic mutations from a diverse viral gene pool. The sudden drop in respiratory virus transmission may have affected the genetic diversity of many viral species by creating an evolutionary bottleneck, but the lasting effects of this evolutionary pressure will vary and will be impacted by many factors, such as changing population-level susceptibility, the introduction of strains through increased international travel and the development of novel vaccines.
Impact of changes in viral transmission
Both baseline pre-pandemic genetic diversity and specific viral characteristics may influence a virus’s ability to resist the selection pressure associated with NPI use. RSV, for example, circulates in the community as two subtypes, RSV-A and RSV-B, that can alternate in predominance in many regions. In Australia, both subtypes frequently co-circulate with similar prevalence 130 . Before the emergence of SARS-CoV-2, RSV genomic diversity was maintained by sources both within and outside Australia 136 . However, genomic analyses of samples obtained before and after the onset of the COVID-19 pandemic showed both a change in the RSV subtype predominance (with RSV-A predominating after community NPIs were implemented) and a reduction in RSV-A sublineages and the emergence of novel lineages, which were genomically linked to frequent domestic importations as well as rarer importations from Washington state in the USA 130 . Whether similar changes in RSV subtype predominance or genomic diversity were experienced in other regions is not known, and subsequent analyses will be needed to understand whether regional RSV diversity could be restored as global travel increases.
By contrast, limited genomic analyses of rhinoviruses suggested a different outcome during the COVID-19 pandemic. Rhinovirus includes several species that are genetically diverse and are able to continuously circulate in the community despite NPIs. Although differentiating rhinovirus types through genomic sequencing is not always possible, the predominance of rhinovirus species in South Korea did not seem to change during the COVID-19 pandemic despite community SARS-CoV-2 mitigation efforts 57 .
Similarly, although there was an initial reduction in the detection of seasonal human coronavirus throughout 2020, epidemics did occur early in 2021 in the USA as all four seasonal human coronavirus species reappeared, coinciding with the relaxing of NPIs later in the COVID-19 pandemic 137 . Epidemic peaks in early 2021 occurred at different time points, differing by geographic region and viral species, with most regions experiencing increased prevalence of seasonal human coronavirus that continued throughout early summer 137 . Data are limited on the effects of the COVID-19 pandemic on seasonal human coronavirus genomic diversity. One study comparing the genomic diversity of seasonal human coronavirus infections in homeless shelters before and during the COVID-19 pandemic showed similar diversity between the two periods 138 .
Impact of changes in immunity
Changes in immunity may also influence the evolution of some viruses. For influenza viruses, for instance, population-level immunity is developed from prior infections and vaccination. With the decline in levels of circulating influenza viruses, lower vaccination coverage and antigenic drift , defined as small changes in the viral genome associated with errors in viral replication, leading to vaccine subtype mismatch 39 , 139 , waning humoral immunity , as defined by lower antibody titres, may make infection more likely, leading to more infections, increased viral replication and additional opportunities for mutations leading to emergence of new variants. It is also possible that in locations where influenza vaccination is not widely administered, waning immunity from recent infections can result in high infection attack rates despite limited influenza virus diversity. Moreover, the strain selection process for the influenza vaccine depends on an evaluation of humoral immunity, specifically haemagglutinin inhibition antibody titres against the circulating virus strains from influenza sentinel surveillance sites in the Northern Hemisphere and the Southern Hemisphere. Low levels of circulating influenza virus may also create challenges for the annual vaccine strain selection process because the smaller number of characterized viruses identified through surveillance makes it more difficult to predict the predominant influenza virus strains for the upcoming season for inclusion in the vaccine, with unknown effect on influenza vaccine effectiveness.
Impact of travel
International travel, which has facilitated the spread of respiratory viruses, may also influence the evolution of certain viruses. Global circulation and seasonal epidemic patterns of influenza virus vary by species and type. Influenza A(H3N2) virus continuously circulated in a complex network of regional epidemics in eastern Asia and Southeast Asia that subsequently facilitated the seeding of temperate regions outside this area, leading to annual seasonal epidemics 140 .
By contrast, during the pandemic, influenza A(H1N1) virus and influenza B virus experienced relatively slower rates of evolution and were less reliant on viral seeding from Asia, with variants continuing to circulate across multiple seasons 141 . It is possible that changes in travel behaviour during the COVID-19 pandemic may have had differential effects on viral species and types. The resumption of global travel is likely to facilitate the spread and evolution of influenza viruses and other respiratory viruses by enhancing the diversity of the viral genetic pool.
The broad application of genomic sequencing to study SARS-CoV-2 molecular epidemiology has enhanced our understanding of respiratory virus evolution. Expanded sequencing of non-SARS-CoV-2 viruses will be useful to monitor viral diversity and evolutionary changes during and after the COVID-19 pandemic, and with the introduction of vaccines, antiviral, and monoclonal antibodies for prevention and treatment.
Changes in the influenza B/Yamagata virus lineage and observations from the influenza A(H1N1)pdm09 pandemic
One evolutionary change observed during the COVID-19 pandemic is the possible extinction of the influenza B/Yamagata virus lineage, with no confirmed detections from March 2020 onward 142 . The 2019–2020 influenza season in the USA was characterized by influenza B/Victoria virus predominance, with a later predominance of influenza A(H1N1)pdm09 virus 143 . During the US 2020–2021 season, low levels of influenza-positive test results were reported, with influenza B virus predominating 144 . Influenza virus activity resumed during 2021–2022 but was lower than in most seasons before the COVID-19 pandemic.
Interestingly, Nextstrain last reported influenza B/Yamagata lineage virus in March 2020 (ref. 145 ). Similarly, FluNet, a WHO global influenza virological surveillance tool started in 1997 through the Global Influenza Surveillance and Response System, has shown a dramatic reduction in influenza B/Yamagata lineage virus detections reported, with none confirmed by sequencing after March 2020 146 (Fig. 2 ). Ongoing global influenza surveillance will determine whether the influenza B/Yamagata virus lineage is no longer circulating and whether this viral antigen no longer needs to be included in the composition of quadrivalent influenza vaccines used worldwide.
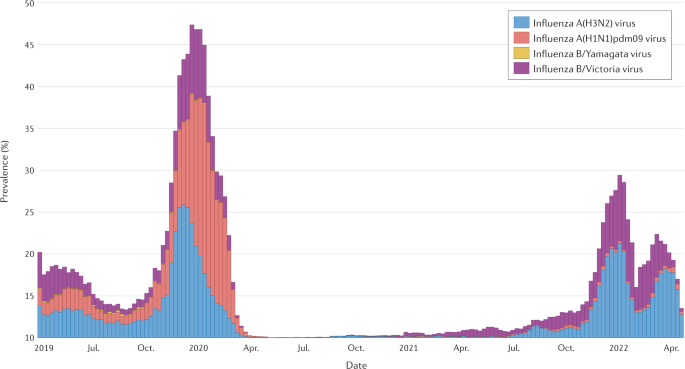
The prevalence of influenza A(H3N2) virus, influenza A(H1N1)pdm09 virus, influenza B/Yamagata virus and influenza B/Victoria virus is shown. The date range of the data extends from 2019 epidemiological week 17 to 2022 epidemiological week 16. The last confirmed report of influenza B/Yamagata virus was in March 2020 (ref. 146 ). Adapted with permission from ref. 146 , WHO.
Recent influenza pandemics offer few clues as to how COVID-19 will affect the circulation of seasonal influenza viruses. In 2009, the emergence of the pandemic influenza A(H1N1)pdm09 virus was associated with a marked decline in seasonal influenza A and influenza B viruses 147 and replacement of seasonal influenza A(H1N1) virus in subsequent seasons 148 . Although low numbers of circulating seasonal influenza viruses were reported while COVID-19 pandemic NPIs were in place, the longer-term effects of the COVID-19 pandemic may not be apparent until future influenza seasons.
Conclusions
The COVID-19 pandemic has led to unprecedented societal and human behaviour changes that have altered the community circulation of non-SARS-CoV-2 respiratory viruses. Although changes in testing priorities and available resources may have influenced the earliest reports of non-SARS-CoV-2 respiratory virus activity, subsequent studies across multiple geographic locations have shown consistent reductions in the activity of most respiratory virus species. However, as the COVID-19 pandemic progressed, there were increasing differences in the application of NPIs and community circulation of SARS-CoV-2 that led to variability in the return of some respiratory viruses. Most notably, influenza virus activity, including the clinical burden of disease and paediatric mortality, remained low through the 2020–2021 season before influenza virus activity increased in 2021–2022. While influenza A and influenza B viruses have begun circulating more widely, influenza B/Yamagata viruses have hardly been detected, and ongoing surveillance will inform whether these viruses might be extinct. Detections of RSV infections initially remained low, with the resurgence of interseasonal circulation in many locations since 2021. There is also evidence that influenza virus diversity and RSV diversity have been reduced as a result of the COVID-19 pandemic, but whether these changes are maintained requires continued surveillance and genomic analyses.
Unlike many of the enveloped viruses, non-enveloped viruses, including rhinovirus, demonstrated remarkable persistence through NPIs, with early evidence showing similar species circulation before and during the COVID-19 pandemic. In part, the persistence of non-enveloped viruses despite NPI implementation may be due to prolonged shedding, their greater diversity and their persistence on environmental surfaces.
These collective findings and lessons learned during the COVID-19 pandemic have important implications for pandemic preparedness. Although the changes in circulation of non-SARS-CoV-2 respiratory viruses cannot be attributed to any one factor, the near-simultaneous implementation of NPIs at individual, community, environmental and country levels, in addition to behavioural changes, seems to have been an important contributor to reducing community circulation, decreasing the associated health care burden and blunting the evolution of non-SARS-CoV-2 respiratory viruses. As such, these collective NPI strategies and global collaboration will be important public health considerations for the next pandemic.
Collaborators, C.-E. M. Estimating excess mortality due to the COVID-19 pandemic: a systematic analysis of COVID-19-related mortality, 2020–21. Lancet 399 , 1513–1536 (2022).
Article Google Scholar
World Health Organization. Non-pharmaceutical public health measures for mitigating the risk and impact of epidemic and pandemic influenza https://www.who.int/publications/i/item/non-pharmaceutical-public-health-measuresfor-mitigating-the-risk-and-impact-of-epidemic-and-pandemic-influenza (2019).
Pan American Health Organization. Non-pharmaceutical interventions (NPIs): actions to limit the spread of the pandemic in your municipality https://www.paho.org/disasters/dmdocuments/RespToolKit_11_Tool%2004_NonPharmaceuticalInterventions(NPIs).pdf (2004).
Qualls, N. et al. Community mitigation guidelines to prevent pandemic influenza - United States, 2017. MMWR Recomm. Rep. 66 , 1–34 (2017).
Article PubMed PubMed Central Google Scholar
Bish, D. R., Bish, E. K., El-Hajj, H. & Aprahamian, H. A robust pooled testing approach to expand COVID-19 screening capacity. PLoS ONE 16 , e0246285 (2021).
Article CAS PubMed PubMed Central Google Scholar
Sullivan, S. G. et al. Where has all the influenza gone? The impact of COVID-19 on the circulation of influenza and other respiratory viruses, Australia, March to September 2020. Eur. Surveill. https://doi.org/10.2807/1560-7917.ES.2020.25.47.2001847 (2020).
Piret, J. & Boivin, G. Viral interference between respiratory viruses. Emerg. Infect. Dis. 28 , 273–281 (2022).
Centers for Disease Control and Prevention. Weekly U.S. influenza surveillance report: FluView https://www.cdc.gov/flu/weekly/index.htm (2022).
Centers for Disease Control and Prevention. The National Respiratory and Enteric Virus Surveillance System (NREVSS) https://www.cdc.gov/surveillance/nrevss/index.html (2022).
World Health Organization. Global Influenza Programme https://www.who.int/tools/flunet (2022).
Centers for Disease Control and Prevention. COVID Data Tracker https://covid.cdc.gov/covid-data-tracker/#cases-deaths-testing-trends (2022).
Johns Hopkins University Coronavirus Resource Center. COVID-19 United States cases 2020 https://coronavirus.jhu.edu/us-map (2020).
World Health Organization. WHO Coronavirus (COVID-19) Dashboard https://covid19.who.int/ (2022).
Chu, H. Y. et al. The Seattle Flu Study: a multiarm community-based prospective study protocol for assessing influenza prevalence, transmission and genomic epidemiology. BMJ Open 10 , e037295 (2020).
State of Washington: Office of the Governor. Proclamation by the Governor Amending Proclamation 20-05: Stay Home-Stay Healthy https://www.governor.wa.gov/sites/default/files/proclamations/20-25%20Coronovirus%20Stay%20Safe-Stay%20Healthy%20%28tmp%29%20%28002%29.pdf (2020).
Seattle Flu Alliance. Pathogens https://seattleflu.org/pathogens (2022).
Laurie, K. L. & Rockman, S. Which influenza viruses will emerge following the SARS-CoV-2 pandemic? Influenza Other Respir. Viruses 15 , 573–576 (2021).
Olsen, S. J. et al. Decreased influenza activity during the COVID-19 pandemic - United States, Australia, Chile, and South Africa, 2020. MMWR Morb. Mortal. Wkly. Rep. 69 , 1305–1309 (2020).
Feng, L. et al. Impact of COVID-19 outbreaks and interventions on influenza in China and the United States. Nat. Commun. 12 , 3249 (2021).
Hills, T., Kearns, N., Kearns, C. & Beasley, R. Influenza control during the COVID-19 pandemic. Lancet 396 , 1633–1634 (2020).
Hay, A. J. & McCauley, J. W. The WHO global influenza surveillance and response system (GISRS) — a future perspective. Influenza Other Respir. Viruses 12 , 551–557 (2018).
World Health Organization. Global Influenza Surveillance and Response System (GISRS) https://www.who.int/initiatives/global-influenza-surveillance-and-response-system (2022).
Centers for Disease Control and Prevention. Fluview: flu activity & surveillance https://www.cdc.gov/flu/weekly/fluactivitysurv.htm (2022).
Roman, P. C. et al. Influenza vaccinations during the COVID-19 pandemic - 11 U.S. jurisdictions, September–December 2020. MMWR Morb. Mortal. Wkly. Rep. 70 , 1575–1578 (2021).
Weycker, D. et al. Population-wide benefits of routine vaccination of children against influenza. Vaccine 23 , 1284–1293 (2005).
Article PubMed Google Scholar
Anderson, E. J., Daugherty, M. A., Pickering, L. K., Orenstein, W. A. & Yogev, R. Protecting the community through child vaccination. Clin. Infect. Dis. 67 , 464–471 (2018).
Cohen, S. A., Chui, K. K. & Naumova, E. N. Influenza vaccination in young children reduces influenza-associated hospitalizations in older adults, 2002–2006. J. Am. Geriatr. Soc. 59 , 327–332 (2011).
Tinoco, Y. O. et al. Burden of Influenza in 4 ecologically distinct regions of Peru: household active surveillance of a community cohort, 2009–2015. Clin. Infect. Dis. 65 , 1532–1541 (2017).
Cohen, C. et al. Asymptomatic transmission and high community burden of seasonal influenza in an urban and a rural community in South Africa, 2017-18 (PHIRST): a population cohort study. Lancet Glob. Health 9 , e863–e874 (2021).
Fogel, B., Schaefer, E. W. & Hicks, S. D. Early influenza vaccination rates decline in children during the COVID-19 pandemic. Vaccine 39 , 4291–4295 (2021).
Olsen, S. J. et al. Changes in influenza and other respiratory virus activity during the COVID-19 pandemic - United States, 2020–2021. MMWR Morb. Mortal. Wkly. Rep. 70 , 1013–1019 (2021).
Park, K. Y., Seo, S., Han, J. & Park, J. Y. Respiratory virus surveillance in Canada during the COVID-19 pandemic: an epidemiological analysis of the effectiveness of pandemic-related public health measures in reducing seasonal respiratory viruses test positivity. PLoS ONE 16 , e0253451 (2021).
Arellanos-Soto, D. et al. Decline in influenza cases in Mexico after the implementation of public health measures for COVID-19. Sci. Rep. 11 , 10730 (2021).
Akhtar, Z. et al. Seasonal influenza during the COVID-19 pandemic in Bangladesh. PLoS ONE 16 , e0255646 (2021).
Li, Z. J. et al. Broad impacts of COVID-19 pandemic on acute respiratory infections in China: an observational study. Clin. Infect. Dis. https://doi.org/10.1093/cid/ciab942 (2021).
Liu, P. et al. Impact of COVID-19 pandemic on the prevalence of respiratory viruses in children with lower respiratory tract infections in China. Virol. J. 18 , 159 (2021).
Huang, W. et al. Epidemiological and virological surveillance of seasonal influenza viruses-China, 2020–2021. China CDC Wkly. 3 , 918–922 (2021).
World Health Organization. Weekly Epidemiological Record (WER), 25 March 2022, Vol. 97, No. 12 , https://reliefweb.int/report/world/weekly-epidemiological-record-wer-25-march-2022-vol-97-no-12-pp109-132-enfr (2022).
Dhanasekaran, V. et al. Human seasonal influenza under COVID-19 and the potential consequences of influenza lineage elimination. Nat. Commun. 13 , 1721 (2022).
Ferguson, N. M. et al. Strategies for mitigating an influenza pandemic. Nature 442 , 448–452 (2006).
Viboud, C. et al. Risk factors of influenza transmission in households. Br. J. Gen. Pract. 54 , 684–689 (2004).
PubMed PubMed Central Google Scholar
Bancej, C. et al. National FluWatch mid-season report, 2021–2022: sporadic influenza activity returns. Can. Commun. Dis. Rep. 48 , 39–45 (2022).
Delahoy, M. J. et al. Influenza A(H3N2) outbreak on a university campus-Michigan, October–November 2021. MMWR Morb. Mortal. Wkly. Rep. 70 , 1712–1714 (2021).
Nott, R., Fuller, T. L., Brasil, P. & Nielsen-Saines, K. Out-of-season influenza during a COVID-19 void in the State of Rio de Janeiro, Brazil: temperature matters. Vaccines 10 , 821 (2022).
Pan American Health Organization. Regional update, influenza. epidemiological week 17 (10 May 2022) https://iris.paho.org/handle/10665.2/55976 (2022).
Australian Government Department of Health. Australian Influenza Surveillance Report https://www1.health.gov.au/internet/main/publishing.nsf/Content/cda-surveil-ozflu-flucurr.htm/$File/flu-08-2022.pdf (2022).
Yeoh, D. K. et al. Impact of coronavirus disease 2019 public health measures on detections of influenza and respiratory syncytial virus in children during the 2020 Australian winter. Clin. Infect. Dis. 72 , 2199–2202 (2021).
Article CAS PubMed Google Scholar
Tempia, S. et al. Decline of influenza and respiratory syncytial virus detection in facility-based surveillance during the COVID-19 pandemic, South Africa, January to October 2020. Eur. Surveill. https://doi.org/10.2807/1560-7917.ES.2021.26.29.2001600 (2021).
Varela, F. H. et al. Absence of detection of RSV and influenza during the COVID-19 pandemic in a Brazilian cohort: likely role of lower transmission in the community. J. Glob. Health 11 , 05007 (2021).
Casalegno, J. S. et al. Characteristics of the delayed respiratory syncytial virus epidemic, 2020/2021, Rhone Loire, France. Eur. Surveill. https://doi.org/10.2807/1560-7917.ES.2021.26.29.2100630 (2021).
Ujiie, M., Tsuzuki, S., Nakamoto, T. & Iwamoto, N. Resurgence of respiratory syncytial virus infections during COVID-19 pandemic, Tokyo, Japan. Emerg. Infect. Dis. 27 , 2969–2970 (2021).
Saravanos, G. L. et al. RSV epidemiology in australia before and during COVID-19. Pediatrics https://doi.org/10.1542/peds.2021-053537 (2022).
Foley, D. A. et al. The interseasonal resurgence of respiratory syncytial virus in Australian children following the reduction of coronavirus disease 2019-related public health measures. Clin. Infect. Dis. 73 , e2829–e2830 (2021).
Olsen, S. J. et al. Changes in influenza and other respiratory virus activity during the COVID-19 pandemic-United States, 2020–2021. Am. J. Transpl. 21 , 3481–3486 (2021).
Article CAS Google Scholar
Centers for Disease Control and Prevention. The National Respiratory and Enteric Virus Surveillance System (NREVSS): RSV census regional trends https://www.cdc.gov/surveillance/nrevss/rsv/region.html (2021).
Cooksey, G. L. S. et al. Severe acute respiratory syndrome coronavirus 2 and respiratory virus sentinel surveillance, California, USA, May 10, 2020–June 12, 2021. Emerg. Infect. Dis. 28 , 9–19 (2022).
Kim, H. M. et al. Impact of coronavirus disease 2019 on respiratory surveillance and explanation of high detection rate of human rhinovirus during the pandemic in the Republic of Korea. Influenza Other Respir. Viruses 15 , 721–731 (2021).
Park, S., Michelow, I. C. & Choe, Y. J. Shifting patterns of respiratory virus activity following social distancing measures for coronavirus disease 2019 in South Korea. J. Infect. Dis. 224 , 1900–1906 (2021).
Kuitunen, I., Artama, M., Haapanen, M. & Renko, M. Rhinovirus spread in children during the COVID-19 pandemic despite social restrictions — a nationwide register study in Finland. J. Med. Virol. 93 , 6063–6067 (2021).
El-Heneidy, A. et al. Respiratory virus detection during the COVID-19 pandemic in Queensland, Australia. Aust. N. Z. J. Public Health 46 , 10–15 (2022).
Eisen, A. K. A. et al. Low circulation of Influenza A and coinfection with SARS-CoV-2 among other respiratory viruses during the COVID-19 pandemic in a region of southern Brazil. J. Med. Virol. 93 , 4392–4398 (2021).
Agrupis, K. A. et al. If not COVID-19 what is it? Analysis of COVID-19 versus common respiratory viruses among symptomatic health care workers in a tertiary infectious disease referral hospital in Manila, Philippines. Trop. Med. Infect. Dis. https://doi.org/10.3390/tropicalmed6010039 (2021).
Kitanovski, S. et al. Rhinovirus prevalence as indicator for efficacy of measures against SARS-CoV-2. BMC Public Health 21 , 1178 (2021).
Dee, K. et al. Human rhinovirus infection blocks severe acute respiratory syndrome Coronavirus 2 replication within the respiratory epithelium: implications for COVID-19 epidemiology. J. Infect. Dis. 224 , 31–38 (2021).
Wu, A., Mihaylova, V. T., Landry, M. L. & Foxman, E. F. Interference between rhinovirus and influenza A virus: a clinical data analysis and experimental infection study. Lancet Microbe 1 , e254–e262 (2020).
Yum, S., Hong, K., Sohn, S., Kim, J. & Chun, B. C. Trends in viral respiratory infections during COVID-19 pandemic, South Korea. Emerg. Infect. Dis. 27 , 1685–1688 (2021).
Takashita, E. et al. Increased risk of rhinovirus infection in children during the coronavirus disease-19 pandemic. Influenza Other Respir. Viruses 15 , 488–494 (2021).
Zhang, K. et al. Rapid disappearance of influenza following the implementation of COVID-19 mitigation measures in Hamilton, Ontario. Can. Commun. Dis. Rep. 47 , 202–209 (2021).
Centers for Disease Control and Prevention. The National Respiratory and Enteric Virus Surveillance System (NREVSS): national trends for common human coronaviruses https://www.cdc.gov/surveillance/nrevss/coronavirus/natl-trends.html (2021).
Ippolito, G. et al. Disappearance of seasonal respiratory viruses in children under two years old during COVID-19 pandemic: a monocentric retrospective study in Milan, Italy. Front. Pediatr. 9 , 721005 (2021).
Russell, K. L. et al. Transmission dynamics and prospective environmental sampling of adenovirus in a military recruit setting. J. Infect. Dis. 194 , 877–885 (2006).
Benschop, K. S. et al. Re-emergence of enterovirus D68 in Europe after easing the COVID-19 lockdown, September 2021. Eur. Surveill. https://doi.org/10.2807/1560-7917.ES.2021.26.45.2100998 (2021).
Dezman, Z. D. W. et al. Masking for COVID-19 is associated with decreased emergency department utilization for non-COVID viral illnesses and respiratory conditions in Maryland. Am. J. Med. 134 , 1247–1251 (2021).
Wood, B. R. et al. Visit trends and factors associated with telemedicine uptake among persons with HIV during the COVID-19 pandemic. Open Forum Infect. Dis. 8 , ofab480 (2021).
Patel, S. Y. et al. Trends in outpatient care delivery and telemedicine during the COVID-19 pandemic in the US. JAMA Intern. Med. 181 , 388–391 (2021).
Kuitunen, I. et al. Effect of social distancing due to the COVID-19 pandemic on the incidence of viral respiratory tract infections in children in Finland during early 2020. Pediatr. Infect. Dis. J. 39 , e423–e427 (2020).
Sun, H. et al. The influence of coronavirus disease 2019 on emergency department visits in Nanjing, China: a multicentre cross-sectional study. Am. J. Emerg. Med. 38 , 2101–2109 (2020).
Jeffery, M. M. et al. Trends in emergency department visits and hospital admissions in health care systems in 5 states in the first months of the COVID-19 pandemic in the US. JAMA Intern. Med. 180 , 1328–1333 (2020).
Adjemian, J. et al. Update: COVID-19 pandemic-associated changes in emergency department visits - United States, December 2020–January 2021. MMWR Morb. Mortal. Wkly. Rep. 70 , 552–556 (2021).
Rodgers, L. et al. Changes in seasonal respiratory illnesses in the United States during the coronavirus disease 2019 (COVID-19) pandemic. Clin. Infect. Dis. 73 , S110–S117 (2021).
Radhakrishnan, L. et al. Pediatric emergency department visits before and during the COVID-19 pandemic - United States, January 2019–January 2022. MMWR Morb. Mortal. Wkly. Rep. 71 , 313–318 (2022).
Angoulvant, F. et al. Coronavirus disease 2019 pandemic: impact caused by school closure and national lockdown on pediatric visits and admissions for viral and nonviral infections-a time series analysis. Clin. Infect. Dis. 72 , 319–322 (2021).
Be’er, M. et al. Unforeseen changes in seasonality of pediatric respiratory illnesses during the first COVID-19 pandemic year. Pediatr. Pulmonol. https://doi.org/10.1002/ppul.25896 (2022).
Perofsky, A. C. et al. The direct and indirect effects of the COVID-19 pandemic on private healthcare utilization in South Africa, March 2020–September 2021. Clin. Infect. Dis. https://doi.org/10.1093/cid/ciac055 (2022).
Onay, Z. R. et al. Did hospital admissions caused by respiratory infections and asthma decrease during the COVID-19 pandemic? Medeni. Med. J. 37 , 92–98 (2022).
COVID-19 Stats. COVID-19* and Influenza(dagger) discharge diagnoses as a percentage of emergency department (ED) visits,(section sign) by year - United States, June 2018–March 2021. MMWR Morb. Mortal. Wkly. Rep. 70 , 573 (2021).
Casalino, E. et al. Analysis of emergency department visits and hospital activity during Influenza season, COVID-19 epidemic, and lockdown periods in view of managing a future disaster risk: a multicenter observational study. Int. J. Environ. Res. Public Health 17 , 8302 (2020).
Stamm, P. et al. Influenza and RSV incidence during COVID-19 pandemic-an observational study from in-hospital point-of-care testing. Med. Microbiol. Immunol. 210 , 277–282 (2021).
Centers for Disease Control and Prevention. Influenza Hospitalization Surveillance Network (FluSurv-NET) https://www.cdc.gov/flu/weekly/influenza-hospitalization-surveillance.htm (2021).
Centers for Disease Control and Prevention. Influenza: US virologic surveillance https://www.cdc.gov/flu/weekly/weeklyarchives2019-2020/Week17.htm (2020).
Centers for Disease Control and Prevention. FluView summary ending on April 24, 2021 https://www.cdc.gov/flu/weekly/weeklyarchives2020-2021/week16.htm (2021).
Song, X. et al. Common seasonal respiratory viral infections in children before and during the coronavirus disease 2019 (COVID-19) pandemic. Infect. Control. Hosp. Epidemiol. https://doi.org/10.1017/ice.2021.430 (2021).
Nolen, L. D. et al. Impact of social distancing and travel restrictions on non-coronavirus disease 2019 (Non-COVID-19) respiratory hospital admissions in young children in rural Alaska. Clin. Infect. Dis. 72 , 2196–2198 (2021).
Stera, G. et al. Impact of SARS-CoV-2 pandemic on bronchiolitis hospitalizations: the experience of an Italian tertiary center. Children https://doi.org/10.3390/children8070556 (2021).
Bermudez Barrezueta, L. et al. Variation in the seasonality of the respiratory syncytial virus during the COVID-19 pandemic. Infection https://doi.org/10.1007/s15010-022-01794-y (2022).
Torres-Fernandez, D., Casellas, A., Mellado, M. J., Calvo, C. & Bassat, Q. Acute bronchiolitis and respiratory syncytial virus seasonal transmission during the COVID-19 pandemic in Spain: a national perspective from the pediatric Spanish Society (AEP). J. Clin. Virol. 145 , 105027 (2021).
Ferrero, F., Ossorio, M. F. & Rial, M. J. The return of RSV during the COVID-19 pandemic. Pediatr. Pulmonol. 57 , 770–771 (2022).
Centers for Disease Control and Prevention. Respiratory Syncytial Virus (RSV) Hospitalization Surveillance Network (RSV-NET) https://www.cdc.gov/rsv/research/rsv-net.html (2021).
Agha, R. & Avner, J. R. Delayed seasonal RSV surge observed during the COVID-19 pandemic. Pediatrics https://doi.org/10.1542/peds.2021-052089 (2021).
Williams, T. C., Sinha, I., Barr, I. G. & Zambon, M. Transmission of paediatric respiratory syncytial virus and influenza in the wake of the COVID-19 pandemic. Eur. Surveill. https://doi.org/10.2807/1560-7917.ES.2021.26.29.2100186 (2021).
Fourgeaud, J. et al. Impact of public health measures on the post-COVID-19 respiratory syncytial virus epidemics in France. Eur. J. Clin. Microbiol. Infect. Dis. 40 , 2389–2395 (2021).
Weinberger Opek, M. et al. Delayed respiratory syncytial virus epidemic in children after relaxation of COVID-19 physical distancing measures, Ashdod, Israel, 2021. Eur. Surveill. https://doi.org/10.2807/1560-7917.ES.2021.26.29.2100706 (2021).
Reicherz, F. et al. Waning immunity against respiratory syncytial virus during the COVID-19 pandemic. J. Infect. Dis. https://doi.org/10.1093/infdis/jiac192 (2022).
Zheng, Z., Pitzer, V. E., Shapiro, E. D., Bont, L. J. & Weinberger, D. M. Estimation of the timing and intensity of reemergence of respiratory syncytial virus following the COVID-19 pandemic in the US. JAMA Netw. Open 4 , e2141779 (2021).
Poole, S., Brendish, N. J., Tanner, A. R. & Clark, T. W. Physical distancing in schools for SARS-CoV-2 and the resurgence of rhinovirus. Lancet Respir. Med. 8 , e92–e93 (2020).
Zhang, R. X. et al. Surges of hospital-based rhinovirus infection during the 2020 coronavirus disease-19 (COVID-19) pandemic in Beijing, China. World J. Pediatr. 17 , 590–596 (2021).
Haddadin, Z. et al. Acute respiratory illnesses in children in the SARS-CoV-2 pandemic: prospective multicenter study. Pediatrics https://doi.org/10.1542/peds.2021-051462 (2021).
Engels, G. et al. Very low incidence of SARS-CoV-2, influenza and RSV but high incidence of rhino-, adeno- and endemic coronaviruses in children with acute respiratory infection in primary care pediatric practices during the second and third wave of the SARS-CoV-2 pandemic. Pediatr. Infect. Dis. J. 41 , e146–e148 (2022).
Thongpan, I., Vichaiwattana, P., Vongpunsawad, S. & Poovorawan, Y. Upsurge of human rhinovirus infection followed by a delayed seasonal respiratory syncytial virus infection in Thai children during the coronavirus pandemic. Influenza Other Respir. Viruses 15 , 711–720 (2021).
Jain, S. et al. Community-acquired pneumonia requiring hospitalization among U.S. children. N. Engl. J. Med. 372 , 835–845 (2015).
Jain, S. et al. Community-acquired pneumonia requiring hospitalization among U.S. adults. N. Engl. J. Med. 373 , 415–427 (2015).
Lai, Y. H., Lin, K. Y., Chang, C. H. & Chang, S. C. Collateral benefit of non-pharmacological interventions against COVID-19 to prevent community-acquired pneumonia in Jin-Shan, New Taipei, April to December 2020. J. Formos. Med. Assoc. 120 , 2195–2196 (2021).
Lastrucci, V. et al. The indirect impact of COVID-19 large-scale containment measures on the incidence of community-acquired pneumonia in older people: a region-wide population-based study in Tuscany, Italy. Int. J. Infect. Dis. 109 , 182–188 (2021).
Nagano, H. et al. Hospitalization of mild cases of community-acquired pneumonia decreased more than severe cases during the COVID-19 pandemic. Int. J. Infect. Dis. 106 , 323–328 (2021).
Yamamoto, T. et al. COVID-19 pandemic and the incidence of community-acquired pneumonia in elderly people. Respir. Investig. 58 , 435–436 (2020).
Huang, C. Pediatric Non-COVID-19 community-acquired pneumonia in COVID-19 pandemic. Int. J. Gen. Med. 14 , 7165–7171 (2021).
Yan, Y., Tomooka, K., Naito, T. & Tanigawa, T. Decreased number of inpatients with community-acquired pneumonia during the COVID-19 pandemic: a large multicenter study in Japan. J. Infect. Chemother. 28 , 709–713 (2022).
Huang, C. The COVID-19 Pandemic and the incidence of the non-COVID-19 pneumonia in adults. Front. Med. 8 , 737999 (2021).
Rybak, A. et al. Shift in clinical profile of hospitalized pneumonia in children in the non-pharmaceutical interventions period during the COVID-19 pandemic: a prospective multicenter study. Front. Pediatr. 10 , 782894 (2022).
Wilder, J. L., Parsons, C. R., Growdon, A. S., Toomey, S. L. & Mansbach, J. M. Pediatric hospitalizations during the COVID-19 pandemic. Pediatrics 146 https://doi.org/10.1542/peds.2020-005983 (2020).
Dahne, T. et al. The impact of the SARS-CoV-2 pandemic on the prevalence of respiratory tract pathogens in patients with community-acquired pneumonia in Germany. Emerg. Microbes Infect. 10 , 1515–1518 (2021).
Hasegawa, K. et al. Respiratory virus epidemiology among us infants with severe bronchiolitis: analysis of 2 multicenter, multiyear cohort studies. Pediatr. Infect. Dis. J. 38 , e180–e183 (2019).
Guitart, C. et al. Bronchiolitis, epidemiological changes during the SARS-CoV-2 pandemic. BMC Infect. Dis. 22 , 84 (2022).
Pelletier, J. H. et al. Trends in US pediatric hospital admissions in 2020 compared with the decade before the COVID-19 Pandemic. JAMA Netw. Open. 4 , e2037227 (2021).
Zee-Cheng, J. E. et al. Changes in pediatric ICU utilization and clinical trends during the coronavirus pandemic. Chest 160 , 529–537 (2021).
Van Brusselen, D. et al. Bronchiolitis in COVID-19 times: a nearly absent disease? Eur. J. Pediatr. 180 , 1969–1973 (2021).
Ferrero, F. & Ossorio, M. F. Is there a place for bronchiolitis in the COVID-19 era? Lack of hospitalizations due to common respiratory viruses during the 2020 winter. Pediatr. Pulmonol. 56 , 2372–2373 (2021).
Friedrich, F. et al. Early impact of social distancing in response to coronavirus disease 2019 on hospitalizations for acute bronchiolitis in infants in Brazil. Clin. Infect. Dis. 72 , 2071–2075 (2021).
Britton, P. N. et al. COVID-19 public health measures and respiratory syncytial virus. Lancet Child. Adolesc. Health 4 , e42–e43 (2020).
Eden, J. S. et al. Off-season RSV epidemics in Australia after easing of COVID-19 restrictions. Nat. Commun. 13 , 2884 (2022).
Shah, S. A., Quint, J. K., Nwaru, B. I. & Sheikh, A. Impact of COVID-19 national lockdown on asthma exacerbations: interrupted time-series analysis of English primary care data. Thorax 76 , 860–866 (2021).
Davies, G. A. et al. Impact of COVID-19 lockdown on emergency asthma admissions and deaths: national interrupted time series analyses for Scotland and Wales. Thorax 76 , 867–873 (2021).
Huh, K. et al. Decrease in hospital admissions for respiratory diseases during the COVID-19 pandemic: a nationwide claims study. Thorax 76 , 939–941 (2021).
Sarc, I. et al. Mortality, seasonal variation, and susceptibility to acute exacerbation of COPD in the pandemic year: a nationwide population study. Ther. Adv. Respir. Dis. 16 , 17534666221081047 (2022).
Ulrich, L., Macias, C., George, A., Bai, S. & Allen, E. Unexpected decline in pediatric asthma morbidity during the coronavirus pandemic. Pediatr. Pulmonol. 56 , 1951–1956 (2021).
Robertson, M. et al. The spatial-temporal dynamics of respiratory syncytial virus infections across the east-west coasts of Australia during 2016-17. Virus Evol. 7 , veab068 (2021).
Centers for Disease Control and Prevention. Census regional trends for common human coronaviruses https://www.cdc.gov/surveillance/nrevss/coronavirus/region.html (2021).
Chow, E. J. et al. The clinical and genomic epidemiology of seasonal human coronaviruses in congregate homeless shelter settings: A repeated cross-sectional study. Lancet Reg. Health Am. 15 , 100348 (2022).
Chung, J. R. et al. Interim estimates of 2021–22 seasonal influenza vaccine effectiveness - United States, February 2022. MMWR Morb. Mortal. Wkly. Rep. 71 , 365–370 (2022).
Russell, C. A. et al. The global circulation of seasonal influenza A (H3N2) viruses. Science 320 , 340–346 (2008).
Bedford, T. et al. Global circulation patterns of seasonal influenza viruses vary with antigenic drift. Nature 523 , 217–220 (2015).
World Health Organization. Recommended composition of influenza virus vaccines for use in the 2022-2023 northern hemisphere influenza season https://cdn.who.int/media/docs/default-source/influenza/who-influenza-recommendations/vcm-northern-hemisphere-recommendation-2022-2023/202202_recommendation.pdf?sfvrsn=5c88e006_13&download=true (2022).
Centers for Disease Control and Prevention. FluView summary ending on September 26, 2020 https://www.cdc.gov/flu/weekly/weeklyarchives2019-2020/Week39.htm (2020).
Centers for Disease Control and Prevention. FluView summary ending on October 2, 2021 https://www.cdc.gov/flu/weekly/weeklyarchives2020-2021/week39.htm (2021).
Nextstrain. Real-time tracking of influenza B/Yam evolution https://nextstrain.org/flu/seasonal/yam/ha/2y (2022).
World Health Organization. FluNet: influenza laboratory surveillance information by the Global Influenza Surveillance and Response System (GISRS) https://apps.who.int/flumart/Default?ReportNo=10 (2022).
Centers for Disease Control and Prevention. FluView 2009-2010 influenza season summary , https://www.cdc.gov/flu/weekly/weeklyarchives2009-2010/09-10summary.htm (2011).
Centers for Disease Control and Prevention. FluView 2010-2011 influenza season summary https://www.cdc.gov/flu/weekly/weeklyarchives2010-2011/10-11summary.htm (2010).
Teng, J. L. L. et al. Substantial decline in invasive pneumococcal disease during Coronavirus disease 2019 pandemic in Hong Kong. Clin. Infect. Dis. 74 , 335–338 (2022).
Brueggemann, A. B. et al. Changes in the incidence of invasive disease due to Streptococcus pneumoniae, Haemophilus influenzae, and Neisseria meningitidis during the COVID-19 pandemic in 26 countries and territories in the Invasive Respiratory Infection Surveillance Initiative: a prospective analysis of surveillance data. Lancet Digit. Health 3 , e360–e370 (2021).
Van Groningen, K. M., Dao, B. L. & Gounder, P. Declines in invasive pneumococcal disease (IPD) during the COVID-19 pandemic in Los Angeles county. J. Infect. https://doi.org/10.1016/j.jinf.2022.05.002 (2022).
Amin-Chowdhury, Z. et al. Impact of the Coronavirus disease 2019 (COVID-19) Pandemic on invasive pneumococcal disease and risk of pneumococcal coinfection with severe acute respiratory syndrome coronavirus 2 (SARS-CoV-2): prospective National Cohort Study, England. Clin. Infect. Dis. 72 , e65–e75 (2021).
Danino, D. et al. Decline in pneumococcal disease in young children during the COVID-19 pandemic in Israel associated with suppression of seasonal respiratory viruses, despite persistent pneumococcal carriage: a prospective cohort study. Clin. Infect. Dis. https://doi.org/10.1093/cid/ciab1014 (2021).
Janapatla, R. P. et al. Serotype transmission dynamics and reduced incidence of invasive pneumococcal disease caused by different serotypes after implementation of non-pharmaceutical interventions during COVID-19 pandemic. Eur. Respir. J. https://doi.org/10.1183/13993003.00978-2021 (2021).
Chan, K. F., Ma, T. F., Ip, M. S. & Ho, P. L. Invasive pneumococcal disease, pneumococcal pneumonia and all-cause pneumonia in Hong Kong during the COVID-19 pandemic compared with the preceding 5 years: a retrospective observational study. BMJ Open 11 , e055575 (2021).
Sarmiento Clemente, A. et al. Decrease in pediatric invasive pneumococcal disease during the COVID-19 pandemic. J. Pediatr. Infect. Dis. Soc. https://doi.org/10.1093/jpids/piac056 (2022).
Wolter, N. et al. High nasopharyngeal pneumococcal density, increased by viral coinfection, is associated with invasive pneumococcal pneumonia. J. Infect. Dis. 210 , 1649–1657 (2014).
Bogaert, D., De Groot, R. & Hermans, P. W. Streptococcus pneumoniae colonisation: the key to pneumococcal disease. Lancet Infect. Dis. 4 , 144–154 (2004).
Valente, C. et al. Decrease in pneumococcal co-colonization following vaccination with the seven-valent pneumococcal conjugate vaccine. PLoS ONE 7 , e30235 (2012).
Dunn, M. G. et al. Impact of 13-valent pneumococcal conjugate vaccine on nasopharyngeal carriage rates of streptococcus pneumoniae in a rural community in the Dominican Republic. J. Infect. Dis. 224 , S237–S247 (2021).
Branche, A. R. et al. Effect of prior vaccination on carriage rates of Streptococcus pneumoniae in older adults: a longitudinal surveillance study. Vaccine 36 , 4304–4310 (2018).
Weiser, J. N., Ferreira, D. M. & Paton, J. C. Streptococcus pneumoniae: transmission, colonization and invasion. Nat. Rev. Microbiol. 16 , 355–367 (2018).
de Steenhuijsen Piters, W. A. A. et al. Interaction between the nasal microbiota and S. pneumoniae in the context of live-attenuated influenza vaccine. Nat. Commun. 10 , 2981 (2019).
Berry, I. et al. Association of influenza activity and environmental conditions with the risk of invasive pneumococcal disease. JAMA Netw. Open 3 , e2010167 (2020).
Kuster, S. P. et al. Evaluation of coseasonality of influenza and invasive pneumococcal disease: results from prospective surveillance. PLoS Med. 8 , e1001042 (2011).
Weinberger, D. M. et al. Pneumococcal disease seasonality: incidence, severity and the role of influenza activity. Eur. Respir. J. 43 , 833–841 (2014).
Russell, C. D. et al. Co-infections, secondary infections, and antimicrobial use in patients hospitalised with COVID-19 during the first pandemic wave from the ISARIC WHO CCP-UK study: a multicentre, prospective cohort study. Lancet Microbe 2 , e354–e365 (2021).
Dirkx, K. K. T. et al. The drop in reported invasive pneumococcal disease among adults during the first COVID-19 wave in the Netherlands explained. Int. J. Infect. Dis. 111 , 196–203 (2021).
Casanova, C., Kuffer, M., Leib, S. L. & Hilty, M. Re-emergence of invasive pneumococcal disease (IPD) and increase of serotype 23B after easing of COVID-19 measures, Switzerland, 2021. Emerg. Microbes Infect. 10 , 2202–2204 (2021).
DeSilva, M. B. et al. Association of the COVID-19 pandemic with routine childhood vaccination rates and proportion up to date with vaccinations across 8 US health systems in the vaccine safety datalink. JAMA Pediatr. 176 , 68–77 (2022).
Choi, Y. H. & Miller, E. Impact of COVID-19 social distancing measures on future incidence of invasive pneumococcal disease in England and Wales: a mathematical modelling study. BMJ Open 11 , e045380 (2021).
Linde, A., Rotzen-Ostlund, M., Zweygberg-Wirgart, B., Rubinova, S. & Brytting, M. Does viral interference affect spread of influenza? Eur. Surveill. 14 , 19354 (2009).
Casalegno, J. S. et al. Rhinoviruses delayed the circulation of the pandemic influenza A (H1N1) 2009 virus in France. Clin. Microbiol. Infect. 16 , 326–329 (2010).
Casalegno, J. S. et al. Impact of the 2009 influenza A(H1N1) pandemic wave on the pattern of hibernal respiratory virus epidemics, France, 2009. Eur. Surveill. 15 , 19485 (2010).
Achten, N. B. et al. Interference between respiratory syncytial virus and human rhinovirus infection in infancy. J. Infect. Dis. 215 , 1102–1106 (2017).
Opatowski, L., Baguelin, M. & Eggo, R. M. Influenza interaction with cocirculating pathogens and its impact on surveillance, pathogenesis, and epidemic profile: a key role for mathematical modelling. PLoS Pathog. 14 , e1006770 (2018).
Nowak, M. D., Sordillo, E. M., Gitman, M. R. & Paniz Mondolfi, A. E. Coinfection in SARS-CoV-2 infected patients: where are influenza virus and rhinovirus/enterovirus? J. Med. Virol. 92 , 1699–1700 (2020).
Essaidi-Laziosi, M. et al. Interferon-dependent and respiratory virus-specific interference in dual infections of airway epithelia. Sci. Rep. 10 , 10246 (2020).
Shinjoh, M., Omoe, K., Saito, N., Matsuo, N. & Nerome, K. In vitro growth profiles of respiratory syncytial virus in the presence of influenza virus. Acta Virol. 44 , 91–97 (2000).
CAS PubMed Google Scholar
Huang, I. C. et al. Influenza A virus neuraminidase limits viral superinfection. J. Virol. 82 , 4834–4843 (2008).
Download references
Acknowledgements
This work was supported in part by the US National Institutes of Health National Institute of Allergy and Infectious Diseases (grant number T32 AI007044 to E.J.C.).
Author information
Authors and affiliations.
Division of Allergy and Infectious Diseases, Department of Medicine, University of Washington, Seattle, WA, USA
Eric J. Chow & Helen Y. Chu
Influenza Division, Centers for Disease Control and Prevention, Atlanta, GA, USA
Timothy M. Uyeki
You can also search for this author in PubMed Google Scholar
Contributions
All authors researched data for the article. All authors substantially contributed to the discussion of the content. All authors contributed to the writing of the manuscript. All authors reviewed/edited the manuscript before submission.
Corresponding author
Correspondence to Helen Y. Chu .
Ethics declarations
Competing interests.
E.J.C. has received honoraria from Providence Health & Services, Seattle, WA, USA, for presentations on COVID-19. H.Y.C. has provided consulting services for Ellume, Pfizer, the Bill and Melinda Gates Foundation, GlaxoSmithKline and Merck. H.Y.C. has received research funding from Gates Ventures and Sanofi Pasteur and has received support and reagents from Ellume and Cepheid outside the submitted work. T.M.U. reports no competing interests.
Peer review
Peer review information.
Nature Reviews Microbiology thanks Aubree Gordon, who co-reviewed with John Kubale, and the other, anonymous, reviewer(s) for their contribution to the peer review of this work.
Additional information
The views expressed are those of the authors and do not necessarily reflect the official policy of the Centers for Disease Control and Prevention.
Publisher’s note
Springer Nature remains neutral with regard to jurisdictional claims in published maps and institutional affiliations.
Supplementary information
Nonspecific symptom-based monitoring used with case definitions to detect early and unusual events or changes from the baseline; requires laboratory testing to provide disease specificity.
(ILI). Fever (temperature of 37.8 °C or greater) and a cough and/or a sore throat.
Disease monitoring by use of specific case definitions at health care facilities to assess disease trends; requires defined a catchment population to generate disease incidence or prevalence rates.
US surveillance system that collects population-level data on laboratory-confirmed influenza-associated hospitalizations across 14 states.
US surveillance system that collects data on respiratory syncytial virus-associated hospitalizations across 12 states each year.
(ARIs). New respiratory symptoms with or without fever caused by infection of the respiratory tract.
(CAP). Pneumonia caused by respiratory pathogens circulating among the community, not health care associated.
Genetic mutations that result in changes to key viral antigens that allow respiratory viruses to escape humoral immunity conferred by prior infection or vaccination.
Adaptive immune response to generate antibodies (antibody-mediated immunity) to the key viral epitopes to prevent infection or reduce the severity of disease.
An open-source scientific platform to visualize pathogen genomic data from GISAID that has included influenza genomic sequences since 2015.
Rights and permissions
Springer Nature or its licensor holds exclusive rights to this article under a publishing agreement with the author(s) or other rightsholder(s); author self-archiving of the accepted manuscript version of this article is solely governed by the terms of such publishing agreement and applicable law.
Reprints and permissions
About this article
Cite this article.
Chow, E.J., Uyeki, T.M. & Chu, H.Y. The effects of the COVID-19 pandemic on community respiratory virus activity. Nat Rev Microbiol 21 , 195–210 (2023). https://doi.org/10.1038/s41579-022-00807-9
Download citation
Accepted : 13 September 2022
Published : 17 October 2022
Issue Date : March 2023
DOI : https://doi.org/10.1038/s41579-022-00807-9
Share this article
Anyone you share the following link with will be able to read this content:
Sorry, a shareable link is not currently available for this article.
Provided by the Springer Nature SharedIt content-sharing initiative
This article is cited by
Chemical inactivation of two non-enveloped viruses results in distinct thermal unfolding patterns and morphological alterations.
- Pankhuri Narula
- Milan Kumar Lokshman
- Manidipa Banerjee
BMC Microbiology (2024)
Viral coinfection in hospitalized patients during the COVID-19 pandemic in Southern Brazil: a retrospective cohort study
- Jaqueline Rhoden
- Andressa Taíz Hoffmann
- Caroline Rigotto
Respiratory Research (2024)
Increased bronchiolitis burden and severity after the pandemic: a national multicentric study
- Sergio Ghirardo
- Nicola Ullmann
- Renato Cutrera
Italian Journal of Pediatrics (2024)
Influenza A, Influenza B, human respiratory syncytial virus and SARSCoV-2 molecular diagnostics and epidemiology in the post COVID-19 era
- Manca Luštrek
Global patterns of rebound to normal RSV dynamics following COVID-19 suppression
- Deus Thindwa
- Daniel M Weinberger
BMC Infectious Diseases (2024)
Quick links
- Explore articles by subject
- Guide to authors
- Editorial policies
Sign up for the Nature Briefing: Microbiology newsletter — what matters in microbiology research, free to your inbox weekly.


IMAGES
VIDEO
COMMENTS
The pandemic marks a stretch on the timeline that tangles with a teetering democracy, a deteriorating planet, the loss of human rights that once felt unshakable.
Having killed more than 7 million people, the COVID-19 pandemic of the early 2020s stands as the most disruptive event to date of the 21st century. Its impact is likely to be felt for generations.
In this essay, we draw on theories of social relationships to examine specific ways in which relational mechanisms key to health and well-being were disrupted by the COVID-19 pandemic. Relational mechanisms refer to the processes between people that lead to change in health outcomes.
How to Write About Coronavirus in a College Essay. Students can share how they navigated life during the coronavirus pandemic in a full-length essay or an optional supplement. By Josh Moody.
The COVID-19 pandemic has led to a dramatic loss of human life worldwide and presents an unprecedented challenge to public health, food systems and the world of work.
How to Write About Coronavirus Using the Special COVID-19 (250-Word) Section on the Common App. Option 1: The Straightforward Way. Option 2: The Slightly More Creative Way. How to Write About Coronavirus Using the (650-Word) Additional Information Section.
Read these 12 moving essays about life during coronavirus. Artists, novelists, critics, and essayists are writing the first draft of history.
A thorough understanding of the epidemiology, pathophysiology and pandemic response efforts to combat COVID-19 is an invaluable lesson to society providing a protocol to fight future pandemics should they occur.
This essay examines key aspects of social relationships that were disrupted by the COVID-19 pandemic. It focuses explicitly on relational mechanisms of health and brings together theory and emerging evidence on the effects of the COVID-19 pandemic to make recommendations for future public health policy and recovery.
The goal of near-simultaneous global implementation of NPIs (Table 1) during the COVID-19 pandemic was to slow down the community transmission of SARS-CoV-2, mitigate the burden of disease...