Navigation auf uzh.ch

Department of Evolutionary Biology and Environmental Studies
Quicklinks und sprachwechsel, main navigation, phd program in ecology.
Completing a PhD research project in the joint University of Zurich (UZH) and Swiss Federal Institute of Technology (ETH) Zurich, PhD Program in Ecology, in one of the internationally acclaimed research groups, enhances a student’s PhD experience. Students of this program are enrolled at either the UZH or the ETH . The program offers research training in the interdisciplinary field of ecology, general skills training for academic and non-academic excellence, and opportunities to interact with other doctoral students. The program includes a curriculum of at least 12 ECTS credits, teaching experience, and is usually completed within three to four years (full-time). It offers yearly graduate schools, courses on subject specific matters and on methods that are of direct use to the work of doctoral students. Additional courses on transferable skills prepare students for professional life, whether this is in an academic institution or not. Research seminars foster international collaborations and the exchange of experiences among doctoral students and experts from different fields of ecology.
___________________________________________________________________
Featured PhD Project: Fall 2024
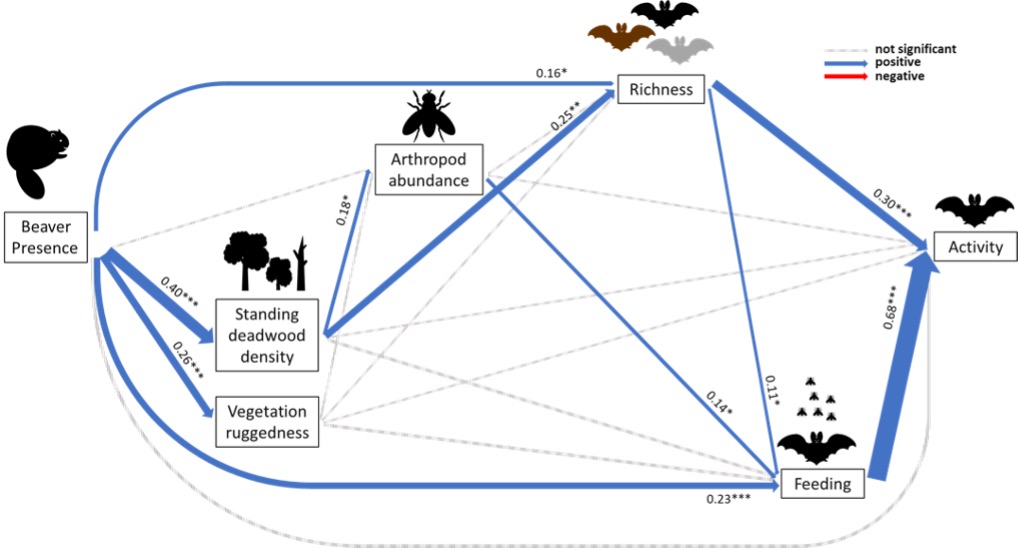
The impact of beavers on blue-green ecosystems Valentin Moser
Beavers influencing bat presence. Beaver influence bat activity directly and indirectly through the change of the environment, leading to an increased habitat heterogeneity and changed arthropod community © Valentin Moser
As ecosystem engineers, beavers (Castor fiber) modify aquatic (blue) and terrestrial (green) ecosystems through building dams and felling trees. The resulting elevated water level and open canopy can benefit different species groups and communities. However, compared to aquatic taxa, the impacts of beaver-engineering on terrestrial taxa and across the blue-green gradient are poorly understood. In my PhD, we sampled biodiversity together with ecosystem properties like soil and water chemistry, as well as ecosystem functions like decomposition rates in 16 systems across Switzerland to better understand how beavers change ecosystems. For bats, our results show correlations between beaver activities and a higher bat presence in these systems. Beaver engineering changes habitat heterogeneity and arthropod abundance, correlated with higher bat species richness, bat activity, and feeding activity. The volume of standing deadwood, a critical resource for bat roosting and foraging, had the strongest correlations with bat presence. Our findings suggest that beavers create heterogeneous landscapes that offer highly diverse ecological niches. By creating environments rich in resources and complexity, beavers may act as natural restoration agents, supporting diverse populations of bats and other species groups. Our results could underscore the importance of integrating beaver management into conservation strategies to restore and enhance aquatic and riparian ecosystems.
Photography for Scientists Science and science communication rely heavily and extensively on photography. In this course organised by the PhD Program in Ecology, student scientists were taught how to be more "visually literate", empowering them to more effectively communicate their science.
Filmmaking for Scientists In this course organised by the PhD Program in Ecology, students learnt how to prepare their own documentary films, including how to deal with camera and lighting, screenwriting/storyboard and film editing. At the end of the workshop, the PhD students prepared a short documentary film.
Weiterführende Informationen

Director: Prof. Dr. Jordi Bascompte Program manager: Dr. Debra Zuppinger-Dingley Department of Evolutionary Biology and Environmental Studies University of Zurich Winterthurerstrasse 190 8057 Zurich Phone : +41 44 635 4778 Office: Y38-L-16 Email: phdecology(at)ieu.uzh.ch
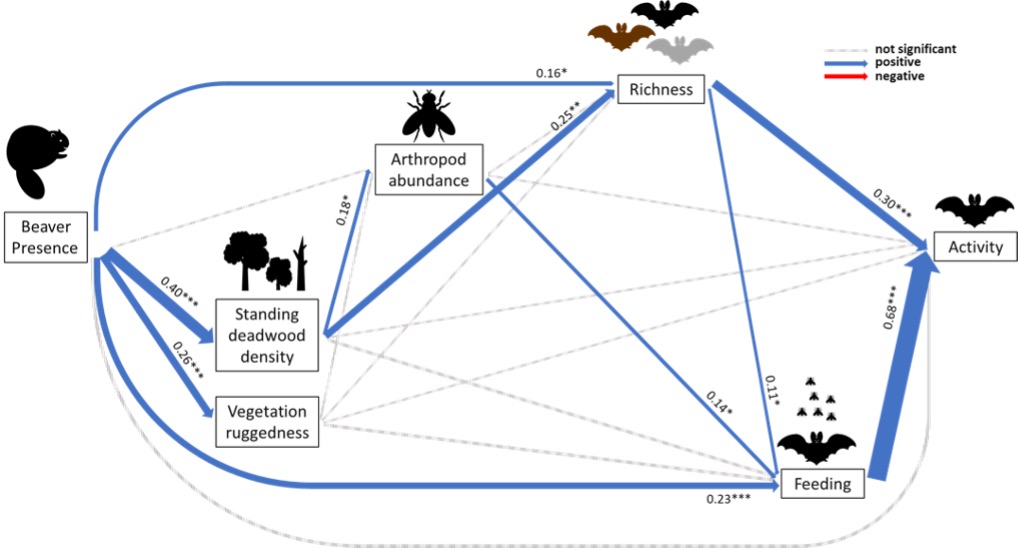
Upcoming courses
Photo credit © Valentin Moser
ProgramEcology HS2024
- Courses fall term 2024
General information
- Poster PhD Program in Ecology (PDF, 55 MB)
Recent News
Open PhD positions at IEU
- Report 2009 (PDF, 1 MB)
Bereichs-Navigation
Unterseiten von phd program in ecology.
- Qualifications and Application
- Program Structure
- Course Overview
- PhD Milestone Overview
- Graduate Summer Schools
- Associated Research Groups
- Student Events
- Featured PhD Projects
7 PhD Degrees in Environmental Studies Ecology in Europe for 2024
- Environmental Studies
Environmental Studies (7)
- Climate Studies (1)
- Coastal Studies (2)
- Human Ecology (1)
- Environmental Management (3)
- Environmental Sciences (9)
- Environmental Sustainability (1)
- Back to main category
- United Kingdom (0)
- Australia (0)
- Bachelor (0)
- Certificate (0)
- Diploma (0)
- Associate of Applied Science (0)
- Associate Degree (0)
- Graduate Certificate (0)
- Associate of Arts (0)
- Advanced Diploma (0)
- Summer Course (0)
- Postgraduate Diploma (0)
- Graduate Diploma (0)
- Foundation Year (0)
- Postgraduate Certificate (0)
- A-level (0)
- Preparatory Program (0)
- Doctor of Education (0)
- Advanced Certificate (0)
- Juris Doctor (0)
- Undergraduate Pathway (0)
- Undergraduate Certificate (0)
- Graduate Pathway (0)
- 2 years (0)
- 3 years (2)
- 4+ years (4)
- Full time (7)
- Part time (2)
- English (7)
- Russian (1)
- Spanish (0)
- Portuguese (0)
- On-Campus (7)
- Distance Learning (0)
- Blended (1)
Popular degree type
Popular study format
Popular education type
Popular locations
PhD Degrees in Environmental Studies Ecology
Individuals who are interested in the effects of climate change and environmental conservation may be interested in studying ecology at a college or university. Ecology programs concentrate their instruction on biodiversity, environmental law, forestry, and pollution in order to help students think about environmental issues on a global scale.
In all, there are over 4000 Higher Education Institutions in Europe offering a wide range of courses at Bachelor, Masters and Doctorate level. With more and more of these organizations offering English as the language of education for at least some of their degree programs, universities in Europe are now of higher quality than ever before. Universities in Europe offer a friendly welcome to foreign students and to give a course of knowledge that meets their profession needs in today’s global demand.
Requirements for the PhD program often involve the student having already obtained a Master’s degree. Additionally, a thesis or dissertation primarily consisting of original academic research must be submitted. In some countries, this work may even need to be defended in front of a panel.
8 PhD Programmes in Environmental Studies Ecology in Europe for 2024
- Environmental Studies
Environmental Studies (8)
- Climate Studies (1)
- Coastal Studies (2)
- Human Ecology (1)
- Environmental Management (4)
- Environmental Sciences (10)
- Environmental Sustainability (1)
- Back to main category
- United Kingdom (1)
- South Africa (0)
- Czech Republic (1)
- Doctor of Education (0)
- 2 years (1)
- 3 years (3)
- 4+ years (3)
- Full time (8)
- Part time (2)
- English (7)
- Spanish (1)
- Russian (1)
- Portuguese (0)
- Italian (0)
- On-Campus (7)
- Distance learning (1)
- Blended (1)
Popular study format
Popular education type
Popular locations
PhD Programmes in Environmental Studies Ecology
Individuals who are interested in the effects of climate change and environmental conservation may be interested in studying ecology at a college or university. Ecology programs concentrate their instruction on biodiversity, environmental law, forestry, and pollution in order to help students think about environmental issues on a global scale.
Europe is, by convention, one of the world's seven continents. Comprising the western most point of Eurasia, Europe is usually divided from Asia by the watershed divides of the Ural and Caucasus Mountains, the Ural River, the Caspian and Black Seas, and the waterways connecting the Black and Aegean Seas.
The PhD is a doctoral degree, specifically called a "doctor of philosophy" degree. This is misleading because PhD holders are not necessarily philosophers (unless they earned their degree in philosophy!). That said, PhD recipients are able to engage in thought experiments, reason about problems, and solve problems in sophisticated ways.
Best Universities for Ecology in Europe
Updated: February 29, 2024
- Art & Design
- Computer Science
- Engineering
- Environmental Science
- Liberal Arts & Social Sciences
- Mathematics
Below is a list of best universities in Europe ranked based on their research performance in Ecology. A graph of 125M citations received by 4.53M academic papers made by 1,358 universities in Europe was used to calculate publications' ratings, which then were adjusted for release dates and added to final scores.
We don't distinguish between undergraduate and graduate programs nor do we adjust for current majors offered. You can find information about granted degrees on a university page but always double-check with the university website.
1. University of Oxford
For Ecology
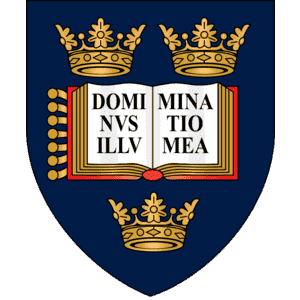
2. University of Cambridge
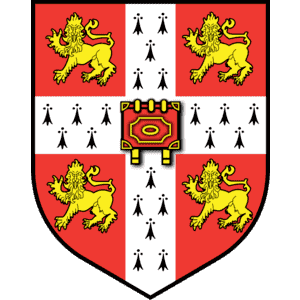
3. Wageningen University
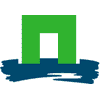
4. Imperial College London
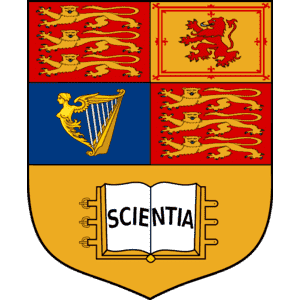
5. University College London
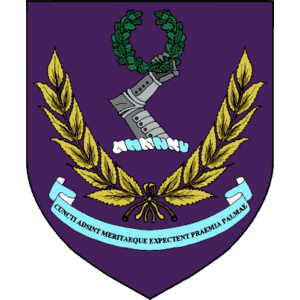
6. Swiss Federal Institute of Technology Zurich
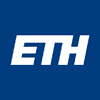
7. Catholic University of Leuven
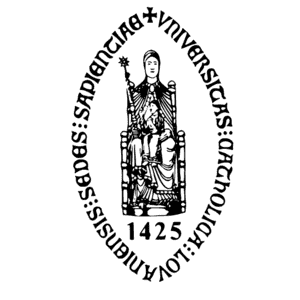
8. Utrecht University
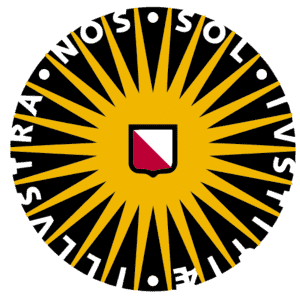
9. University of Copenhagen
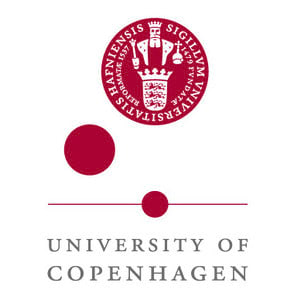
10. Lund University
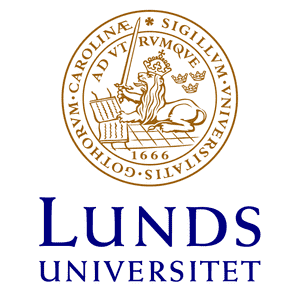
11. Ghent University
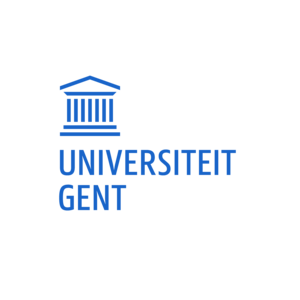
12. Swedish University of Agricultural Sciences
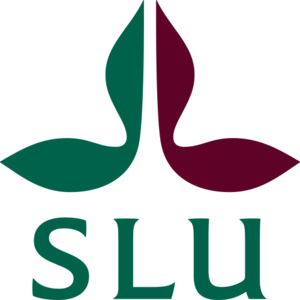
13. University of Edinburgh
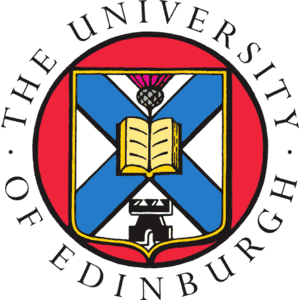
14. University of Helsinki
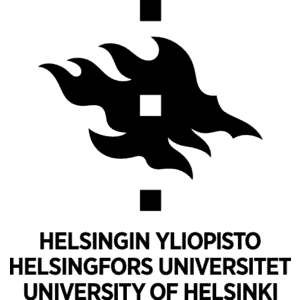
15. Pierre and Marie Curie University
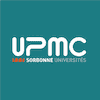
16. Federal Institute of Technology Lausanne
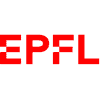
17. Technical University of Denmark
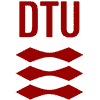
18. University of Manchester
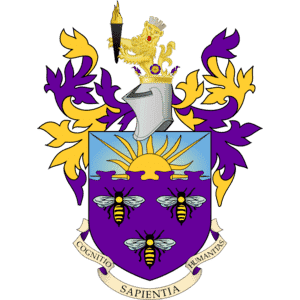
19. University of Bristol
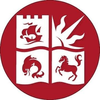
20. Aarhus University
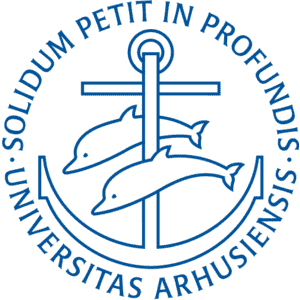
21. University of Sheffield
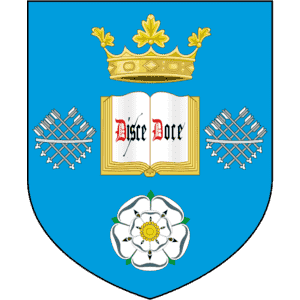
22. Delft University of Technology
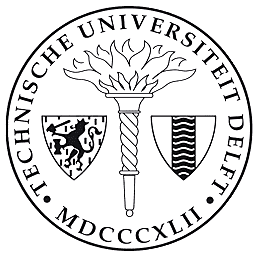
23. University of Zurich
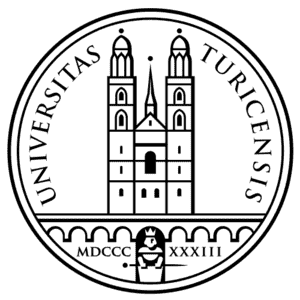
24. University of Leeds
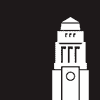
25. Uppsala University
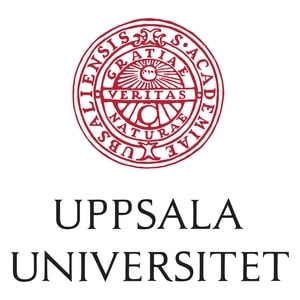
26. Stockholm University
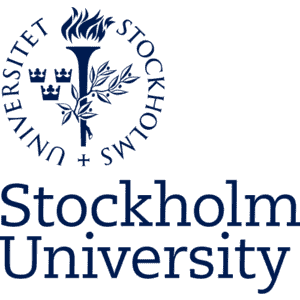
27. University of Amsterdam
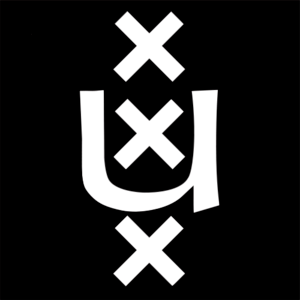
28. University of Nottingham
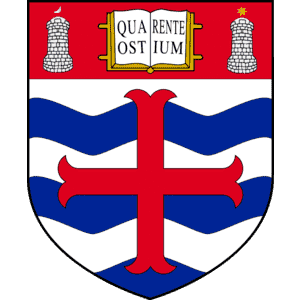
29. Technical University of Munich
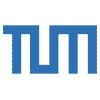
30. University of Groningen
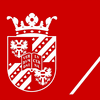
31. University of Birmingham
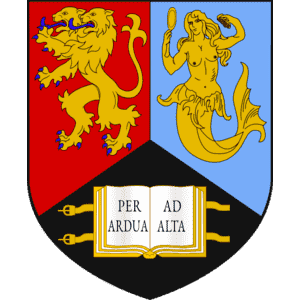
32. University of Gottingen
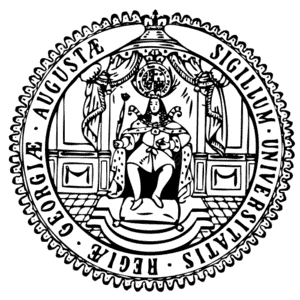
33. University of Southampton
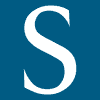
34. University of Reading
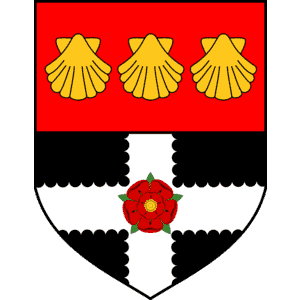
35. University of East Anglia
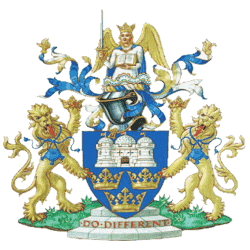
36. University of Liege
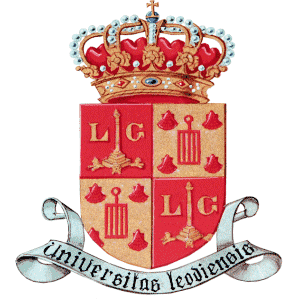
37. University of Oslo
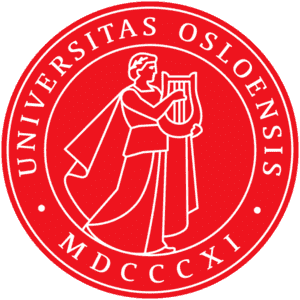
38. University of Vienna
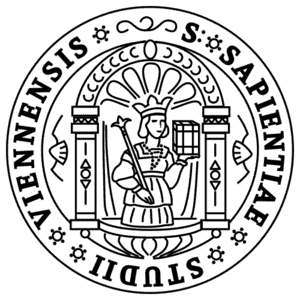
39. Newcastle University
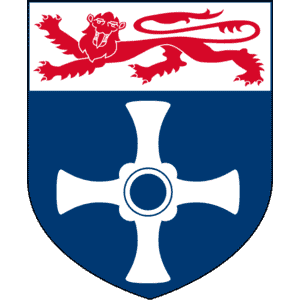
40. University of Exeter
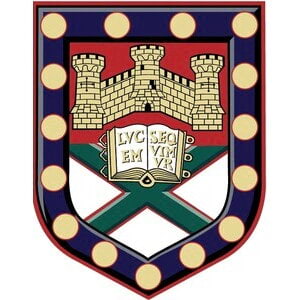
41. University of Liverpool
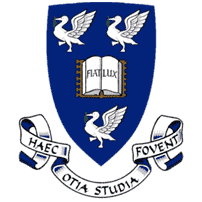
42. Polytechnic University of Bari
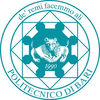
43. University of Gothenburg
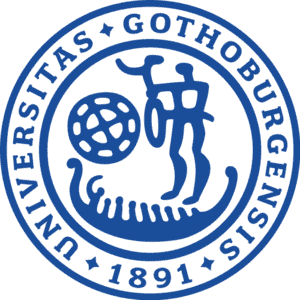
44. University of Bologna
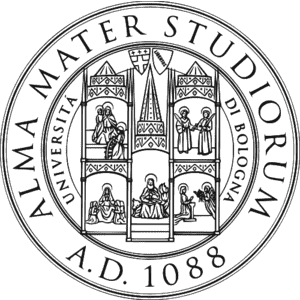
45. Sapienza University of Rome
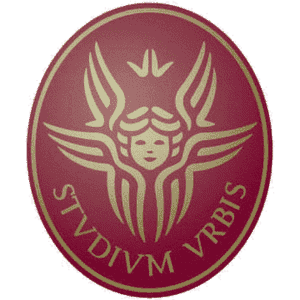
46. University of Lisbon
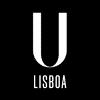
47. University of Padua
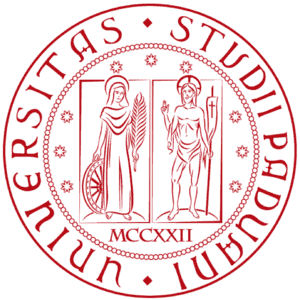
48. University of Aberdeen
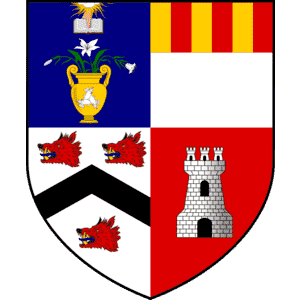
49. University of Barcelona
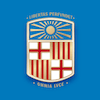
50. University of Glasgow
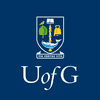
51. Norwegian University of Science and Technology
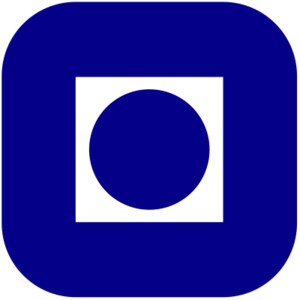
52. University of Bern
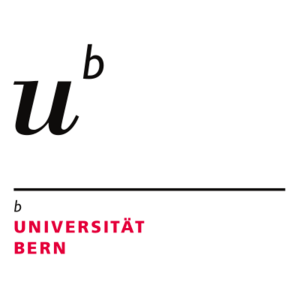
53. University of Hamburg
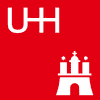
54. Claude Bernard University Lyon 1
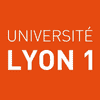
55. University of Bonn
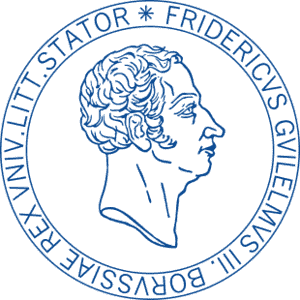
56. Free University Amsterdam
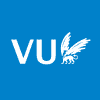
57. Aristotle University of Thessaloniki
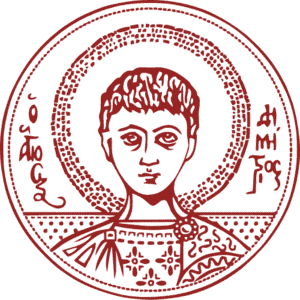
58. University of Porto
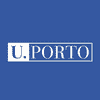
59. Autonomous University of Barcelona
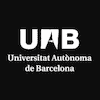
60. Federico II University of Naples
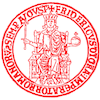
61. King's College London
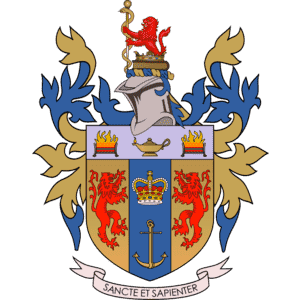

62. Radboud University
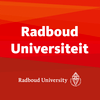
63. University of Montpellier
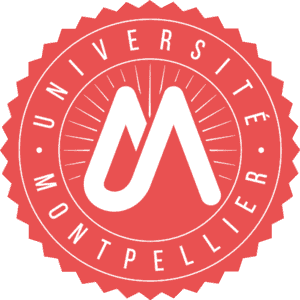
64. University of Milan
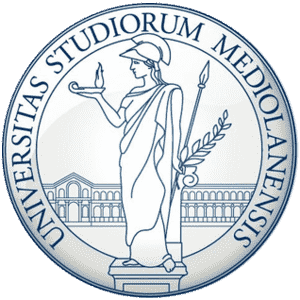
65. University of York
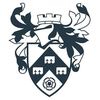
66. Moscow State University
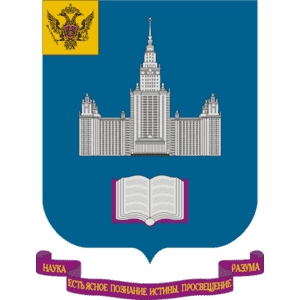
67. Aalborg University
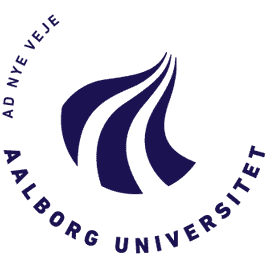
68. Complutense University of Madrid
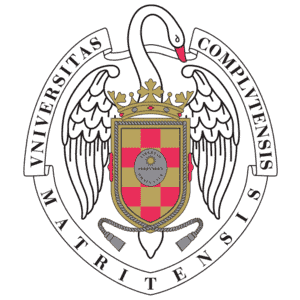
69. Cardiff University
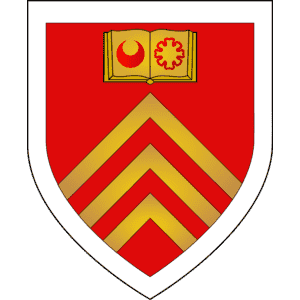
70. Heidelberg University - Germany
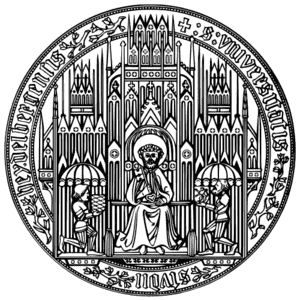
71. Lancaster University
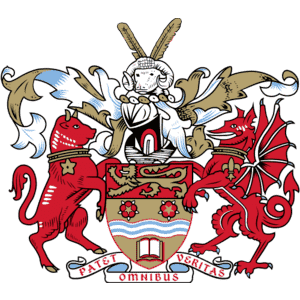
72. Karlsruhe Institute of Technology
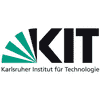
73. University of Sussex
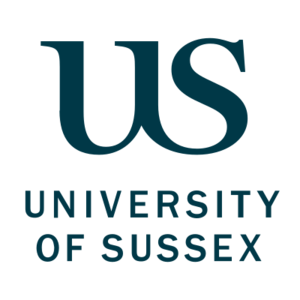
74. University of Munich
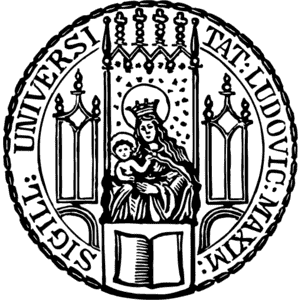
75. Kiel University
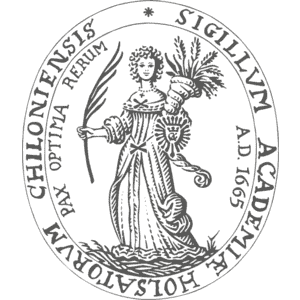
76. University of Bergen
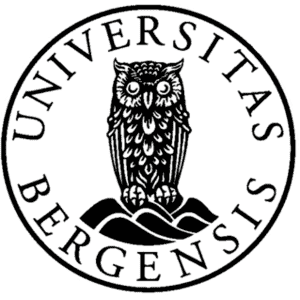
77. Leiden University
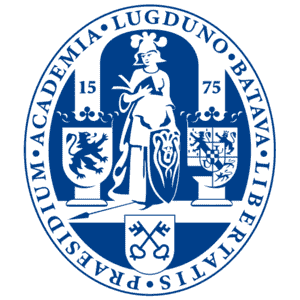
78. Polytechnic University of Milan
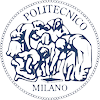
79. University of Florence
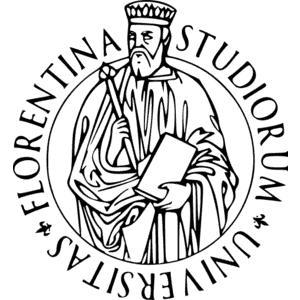
80. RWTH Aachen University
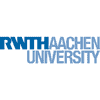
81. KTH Royal Institute of Technology
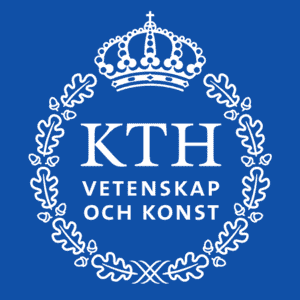
82. Free University of Berlin
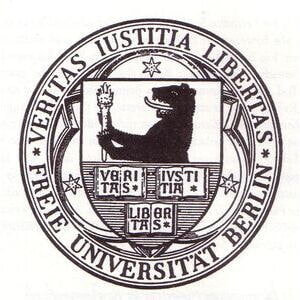
83. University of Antwerp
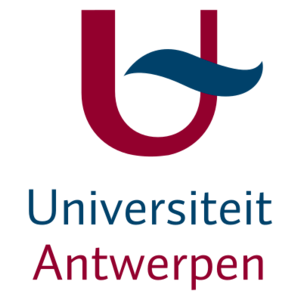
84. University College Dublin
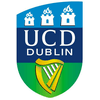
85. Catholic University of Louvain
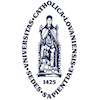
86. Umea University
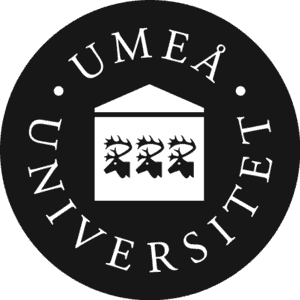
87. University of Freiburg
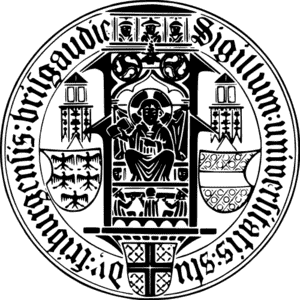
88. University of Valencia
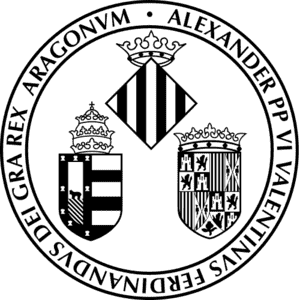
89. Chalmers University of Technology
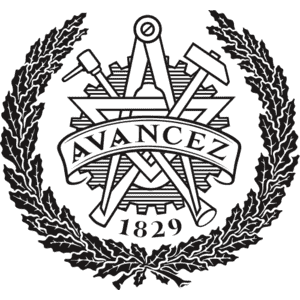
90. University of St Andrews
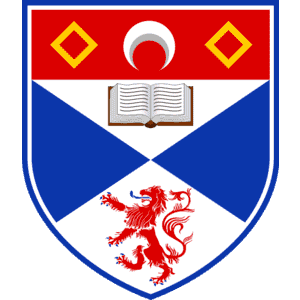
91. Technical University of Berlin
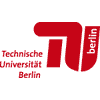
92. University of Granada
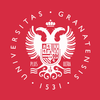
93. Eindhoven University of Technology
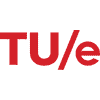
94. University of Pisa
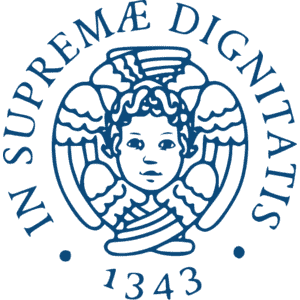
95. University of Warwick
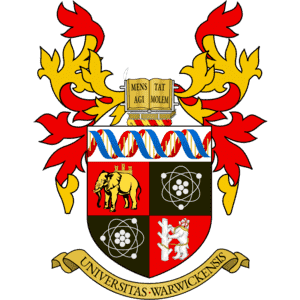
96. Technical University of Catalonia
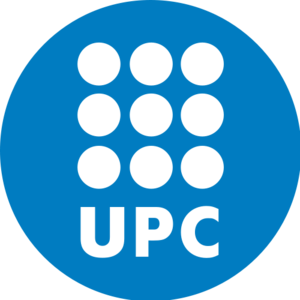
97. University of Bayreuth
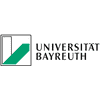
98. University of London
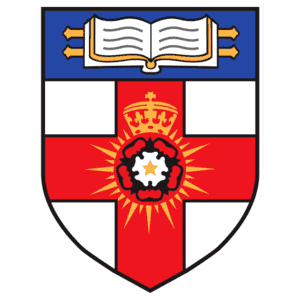
99. Queen Mary University of London
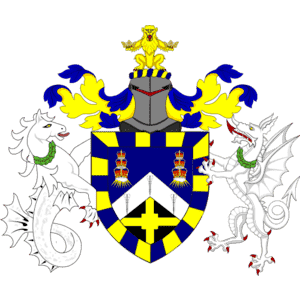
100. Queen's University Belfast
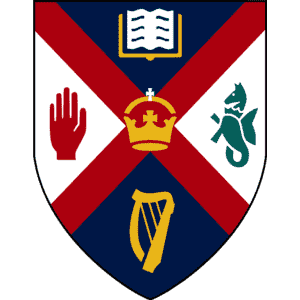
Environmental Science subfields in Europe
We use cookies on reading.ac.uk to improve your experience, monitor site performance and tailor content to you
Read our cookie policy to find out how to manage your cookie settings
This site may not work correctly on Internet Explorer. We recommend switching to a different browser for a better experience.
PhD opportunities
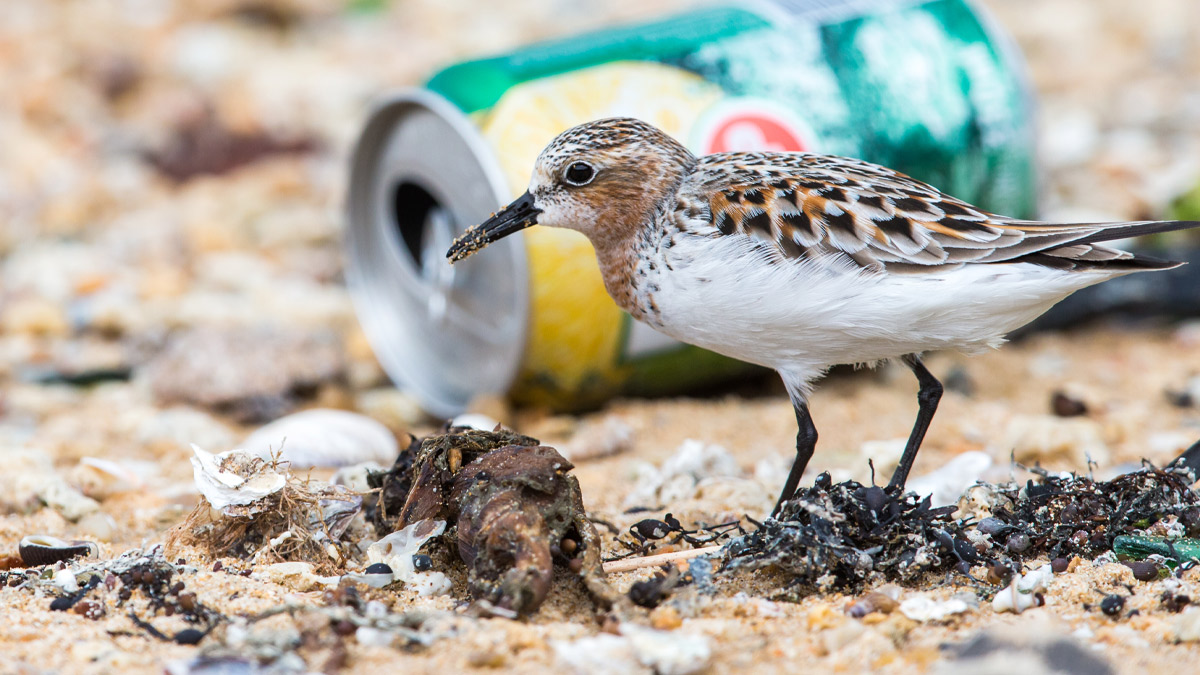
We offer PhD degrees in ecology and evolutionary biology.
Ecologists can research interactions between species and between species and their environments that influence abundance and distribution. Address climate change adaptation, design of habitat networks and agri-environment schemes.
Evolutionary biologists will use modern analytical tools to research the evolution of plants, animals and microbes. We’re developing new methods to incorporate fossils and geographic data into phylogenies, detect natural selection on phenotypes and probe gene network evolution and adaptive landscapes.
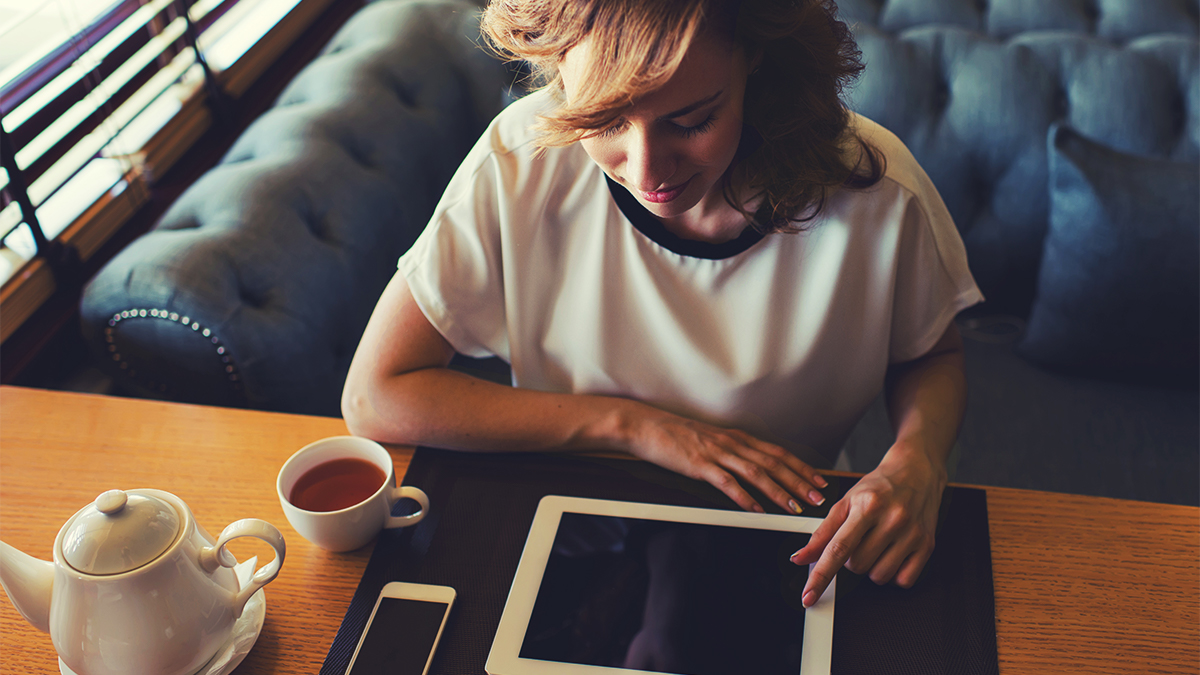
Types of doctoral degree
We offer several routes to a doctoral qualification, so you can find the one that suits you and the topic you wish to study.
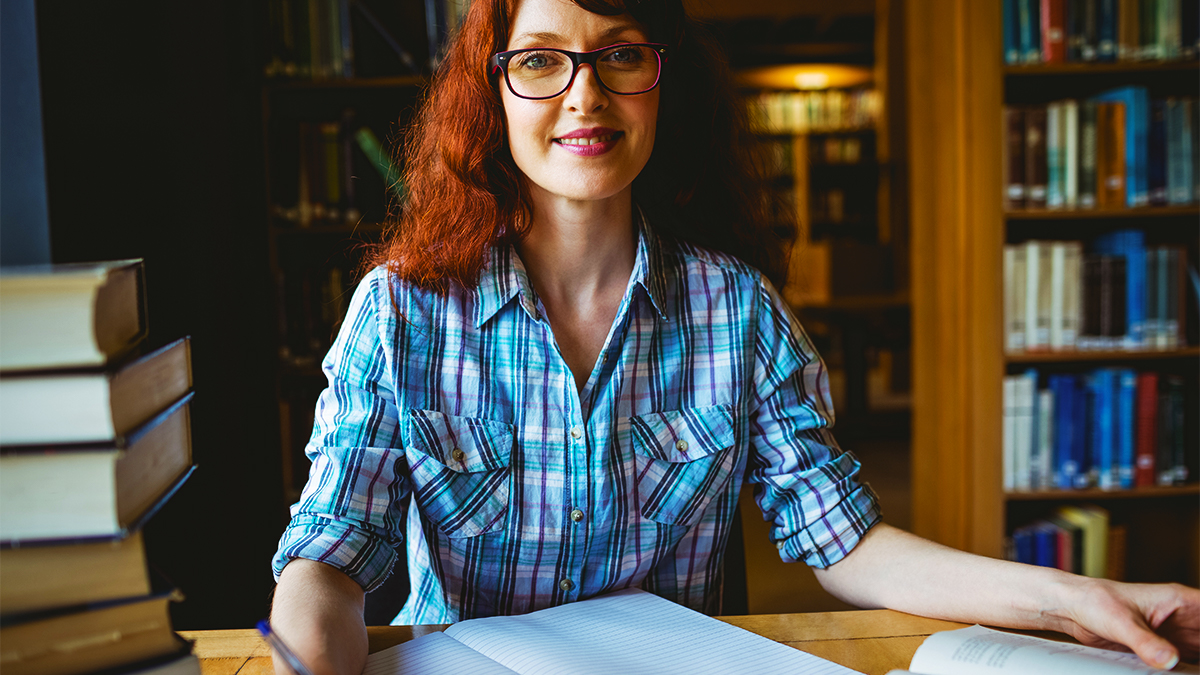
Part-time study
Part-time PhDs are available as well as full-time, so you can choose a mode of study that suits your circumstances.
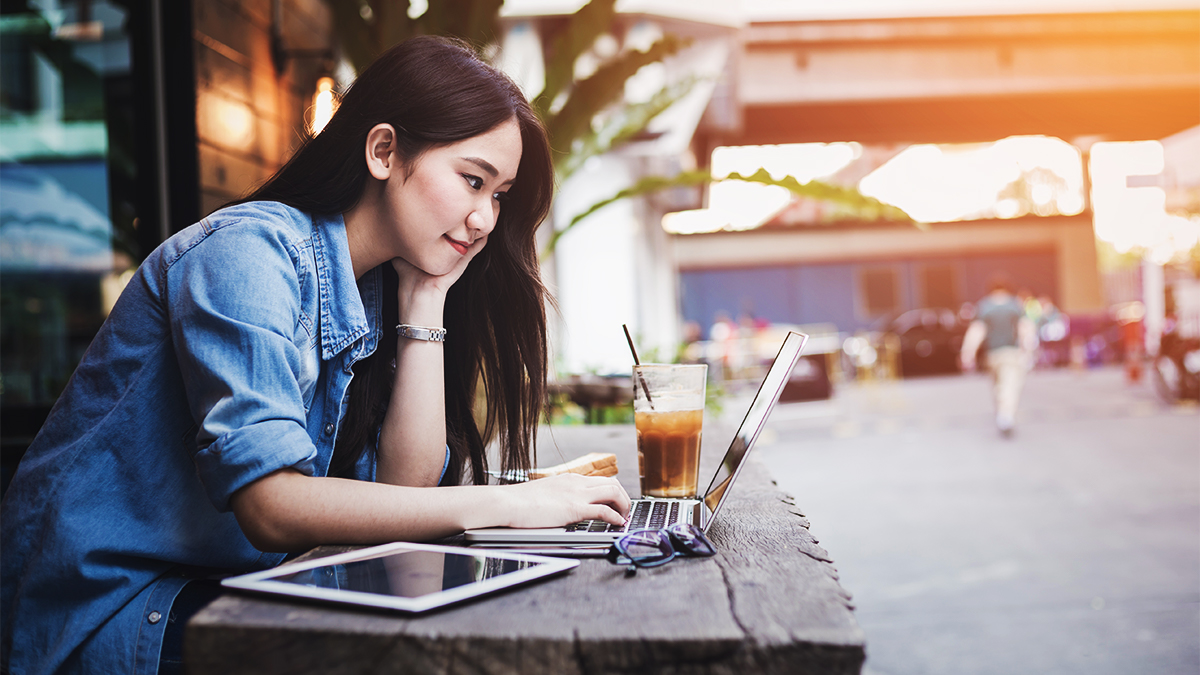
PhD by Distance
Our PhD by Distance programme allows you to benefit from the expertise of a Reading-based supervisor, while conducting your research in a different location.
Find a PhD opportunity that aligns with your interests and career ambitions.
We want to ensure that your time spent with us is as rewarding as possible. To allow you to explore your various options, here's a list of some of currently available PhD opportunities across the School of Biological Sciences.
We do, however, offer many more options, so please contact us for further information. You can propose your own project that aligns with our research. Find out more about how to apply for a PhD .
Take the next step
- How to Apply
- Get a prospectus
- Ask us a question
- Learn about the Doctoral and Researcher College
Numerous Fully Funded PhD Positions Across Europe for International Students

Over 25 Fully Funded PhD Positions Await International Students!
Are you eager to begin an exceptional academic journey in Europe? 🇦🇹, 🇧🇪, 🇩🇰, 🇩🇪, 🇫🇷, 🇬🇧, 🇬🇷, 🇭🇺, 🇮🇪, 🇮🇸, 🇱🇺, 🇳🇱, 🇳🇴, 🇪🇸, 🇨🇭
Your opportunity is here! We are thrilled to announce that more than 25 fully funded PhD positions are available for international students who aspire to pursue their PhD studies at leading European institutions, including:
🇬🇷 Institute of Astrophysics - FORTH
🇦🇹 Graz University of Technology
🇩🇰 University of Copenhagen
🇭🇺 University of Pannonia
🇩🇪 Leibniz-Institut für Analytische Wissenschaften ISAS
🇳🇴 NTNU Norwegian University of Science and Technology
🇮🇸 Reykjavik University
🇳🇱 Stichting Deltares
🇪🇸 IMDEA Networks Institute
🇨🇭 Zürcher Hochschule für angewandte Wissenschaft
🇬🇧 Swansea University
🇱🇺 Luxembourg Institute of Science and Technology
🇳🇴 UiT The Arctic University of Norway
🇧🇪 Université Libre de Bruxelles (ULB)
🇦🇹 AIT Austrian Institute of Technology GmbH
🇩🇪 Fraunhofer ICT
🇫🇷 University of Strasbourg
🇮🇪 University of Limerick
🇦🇹 St. Anna Kinderkrebsforschung
🇳🇴 University of South-Eastern Norway
🇩🇪 Leibniz-Institute for Plant Genetics and Crop Plant Research
🇩🇪 Friedrich-Alexander-Universität Erlangen-Nürnberg
Apply now for the following doctoral (PhD) studies in diverse fields at esteemed European universities:-
PhD Student in Multimessenger observations of TDEs, Institute of Astrophysics - FORTH (Greece)
PhD Student in Engineering Enzymes for the Removal of Toxic C-F Containing Compounds, Graz University of Technology (Austria)
PhD fellowship in computational and mathematical modelling of flow and fracture in ice sheets, University of Copenhagen (Denmark)
PhD Student in Material Science, Physics and Environmental Sciences, University of Pannonia (Hungary)
PhD Student in Analysis of Microscopic BIOMedical Images (AMBIOM), Leibniz-Institut für Analytische Wissenschaften - ISAS - e.V. (Germany)
PhD Student in Compiler Technologies, NTNU Norwegian University of Science and Technology (Norway)
PhD Student in AI-guided Discovery and Prediction of Novel Enzymes in Microbiomes of Different Habitats, Graz University of Technology (Austria)
PhD Student in Climate Change, Finance, and Sustainability, Reykjavik University (Iceland)
PhD Student in marine sustainability and conservation for European waters, Stichting Deltares (Netherlands)
PhD Student in blockchain and peer-to-peer networks, IMDEA Networks Institute (Spain)
PhD Student in Electrochemical double layer and meso-scale modelling, Zürcher Hochschule für angewandte Wissenschaft (Switzerland)
PhD Student in Advanced Optoelectronic & Microelectronic Glass at Swansea University, Swansea University (United Kingdom)
PhD Student in Piezoelectric Sensors, Luxembourg Institute of Science and Technology (Luxembourg)
PhD Fellow in Optics – Quantitative phase microscopy and Tomography, UiT The Arctic University of Norway (Norway)
PhD Student in Transmembrane transport studies in artificial systems, Université Libre de Bruxelles (ULB) (Belgium)
PhD Student in Digital Twins for Transportation, AIT Austrian Institute of Technology GmbH (Austria)
PhD Student in Automated potentio-dynamic electrochemical characterization, Fraunhofer ICT (Germany)
PhD Student in MRI-based cavitation control for ultrasound-induced drug delivery,
University of Strasbourg (France)
PhD Student in Aging Models and Reliability of Extruded HVDC Cables for Offshore Power, NTNU Norwegian University of Science and Technology (Norway)
PhD Student in Catalysis for Renewable Feedstock Valorisation, University of Limerick (Ireland)
PhD Student in Pediatric Cancer Initiation, St. Anna Kinderkrebsforschung e.V. (Austria)
PhD Student in Vertical Multi-Purpose Farming Robotic System, Swansea University (United Kingdom)
PhD Fellow in Cybersecurity, University of South-Eastern Norway (Norway)
PhD Student in the field of Root Anatomy, Leibniz-Institute for Plant Genetics and Crop Plant Research (Germany)
PhD Student in Surface Acoustic Wave Strain Sensor, Luxembourg Institute of Science and Technology (Luxembourg)
PhD Student in digital twins for 6G mobile networks, IMDEA Networks Institute (Spain)
PhD Student in molecular dynamics simulation in mineral processing, NTNU Norwegian University of Science and Technology (Norway)
PhD Student in FAME consortium, Friedrich-Alexander-Universität Erlangen-Nürnberg (Germany)
PhD Fellow in Human factors in remotely operated and autonomous maritime vessels,
UiT The Arctic University of Norway (Norway)
#EURAXESSAfrica #PhD #DoctoralStudy #DoctoralPositions #PhDPositions #PhDStudy #Europe #EU #StudyInEurope #HigherEducation #InternationalStudents #ResearchOpportunities #FORTH #GrazUniversityofTechnology #UniversityofCopenhagen #UniversityofPannonia #LSAS #NTNU #ReykjavikUniversity #StichtingDeltares #IMDEANetworksInstitute #ZürcherHochschulefürangewandteWissenschaft #SwanseaUniversity #LuxembourgInstituteofScienceandTechnology #UiT #ULB #AIT #FraunhoferICT #UniversityofStrasbourg #UniversityofLimerick #St_AnnaKinderkrebsforschung #UniversityofSouthEasternNorway #LeibnizInstituteforPlantGeneticsandCropPlantResearch #FriedrichAlexanderUniversitätErlangenNürnberg
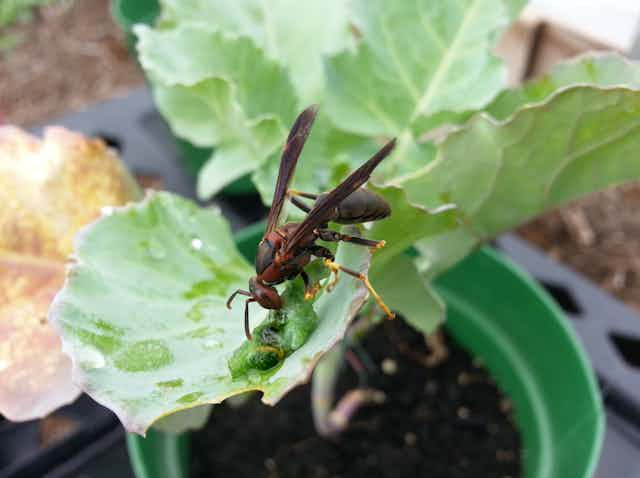
Wasps can be pests in NZ – but they have potential to be pest controllers too
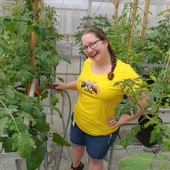
Senior Lecturer in Ecology, University of Otago
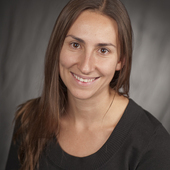
Professor & Chair, Graduate Program in Ecology and Evolutionary Biology, Iowa State University
Disclosure statement
The authors do not work for, consult, own shares in or receive funding from any company or organisation that would benefit from this article, and have disclosed no relevant affiliations beyond their academic appointment.
Iowa State University provides funding as a member of The Conversation US.
University of Otago provides funding as a member of The Conversation AU.
University of Otago provides funding as a member of The Conversation NZ.
View all partners
What good are wasps? It’s a question we hear all the time. And, let’s face it, wasps are routinely demonised .
They interrupt picnics (they love beer and fruit as much as we do). Their sting can be painful or even cause an allergic reaction requiring medical treatment. And a swarm of them flying at you is genuinely terrifying.
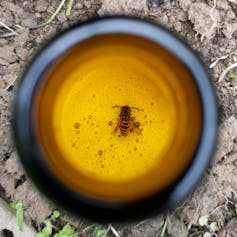
The beech forests in the northern South Island of New Zealand are home to the world’s highest populations of ground-nesting Vespula wasps, also known as the German or common wasp. So it’s not surprising they get a lot of press, and hardly any of it is positive.
But this reputation is not entirely deserved. In fact, some types of wasp have been shown to be useful in crop pest control – something New Zealand’s horticultural industries may benefit from.
Wasps in New Zealand
Foreign wasps arrived in New Zealand around the middle of the 20th century, after queens stowed away in cargo ships from Europe. The new habitat suited their life cycle , and the wasps thrived.
Like honey bees and bumble bees, the two main “social wasp” groups – Polistes (paper wasps), and Vespula (ground-nesting wasps) – aren’t native. But unlike bees, they are active predators of other insects.
This means they compete for food with native birds and lizards, and can also affect the populations of some endemic insect populations (especially in beech forests). They may also periodically terrorise managed beehives. Consequently, there are nationwide efforts to eradicate social wasps in New Zealand.
But these wasps eat the insects that “bug” us too. Evidence has been mounting for more than 40 years that wasps – particularly Polistes , which are major predators of caterpillars – may be effective at controlling crop pests.
Beneficial predators
In our recent study in the Midwestern United States, we moved wasp colonies into a large, screened cage containing broccoli plants. We then added caterpillars and measured how the presence of wasps affected pest consumption of the plants.
The wasps ate all the caterpillars we set out within three hours. They were clever, too, eventually following us as we put the caterpillars on the crops. We also realised some wasps had worked out how to sneak onto our control plants (from which they were meant to be excluded).
We revised our controls and re-ran the study, this time using a different species of Polistes and a different crop, kale. By day six, all caterpillars from our plants were missing.
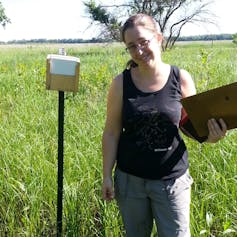
The results were clear. Not only can we move Polistes wasp colonies to a new location, but these paper wasps are active predators of crop pests. When wasps were excluded from the broccoli or kale that caterpillars had been added to, pest damage increased. When wasps were allowed to hunt, the crops prospered.
Like Vespula wasps, Polistes can sting if their nest is disturbed. But unlike Vespula , Polistes build small nests under the eaves of buildings. Their nests are relatively easy to remove and relocate to a better spot.
By relocating Polistes wasps into these boxes, we can move nests into small areas or distribute around a crop field. The boxes also act as a buffer to reduce the chances of disturbing the nest and being stung.
Pest control potential
If you watch wasps long enough, you can observe them carrying pests away from your home garden, local park or farm.
So, could Polistes be harnessed as a pest controller in New Zealand? Our research suggests they hold biocontrol potential for garden pests. But more research is needed to determine how effective that might be, and to ensure they are targeting pests and minimising their impact on native species.
Ours is also not the first study to highlight the benefits of social wasps. Beyond pest control, wasps are effective flower pollinators and help spread yeasts on grapes that improve wine quality. Wasp venoms are now being harnessed as medicines, including as a possible cancer treatment .
Overall, we argue wasps should not be uniformly demonised. They exhibit complex social behaviours to rival anything on Game of Thrones. And they were the original paper manufacturers , turning dry wood, cardboard or paper into pulp with their saliva to create the nest cells where their larvae will grow.
Perhaps one day we can harness them as biocontrol agents targeting crop pests, and reduce reliance on pesticides that can negatively affect beneficial insects such as bees. Like other wasp enthusiasts , we’d like to think their ability to help control pests on valuable crops might one day rehabilitate their reputation.
- New Zealand
- Pest control

Project Manager SSTP

Head of Evidence to Action

Supply Chain - Assistant/Associate Professor (Tenure-Track)

OzGrav Postdoctoral Research Fellow

Casual Facilitator: GERRIC Student Programs - Arts, Design and Architecture
Accessibility Links
- Skip to content
- Skip to search IOPscience
- Skip to Journals list
- Accessibility help

The American Astronomical Society (AAS) , established in 1899 and based in Washington, DC, is the major organization of professional astronomers in North America. Its membership of about 7,000 individuals also includes physicists, mathematicians, geologists, engineers, and others whose research and educational interests lie within the broad spectrum of subjects comprising contemporary astronomy. The mission of the AAS is to enhance and share humanity's scientific understanding of the universe.

The Institute of Physics (IOP) is a leading scientific society promoting physics and bringing physicists together for the benefit of all. It has a worldwide membership of around 50 000 comprising physicists from all sectors, as well as those with an interest in physics. It works to advance physics research, application and education; and engages with policy makers and the public to develop awareness and understanding of physics. Its publishing company, IOP Publishing, is a world leader in professional scientific communications.
A publishing partnership
First Very Long Baseline Interferometry Detections at 870 μ m
Alexander W. Raymond 1,2,3 , Sheperd S. Doeleman 1,2,151 , Keiichi Asada 4 , Lindy Blackburn 1,2 , Geoffrey C. Bower 5,6 , Michael Bremer 7 , Dominique Broguiere 7 , Ming-Tang Chen 4 , Geoffrey B. Crew 8 , Sven Dornbusch 9 , Vincent L. Fish 8 , Roberto García 7 , Olivier Gentaz 7 , Ciriaco Goddi 10,11 , Chih-Chiang Han 4 , Michael H. Hecht 8 , Yau-De Huang 4 , Michael Janssen 18,9 , Garrett K. Keating 2 , Jun Yi Koay 4 , Thomas P. Krichbaum 9 , Wen-Ping Lo 4,12 , Satoki Matsushita 4 , Lynn D. Matthews 8 , James M. Moran 1,2 , Timothy J. Norton 2 , Nimesh Patel 2 , Dominic W. Pesce 1,2 , Venkatessh Ramakrishnan 13,14,15 , Helge Rottmann 9 , Alan L. Roy 9 , Salvador Sánchez 16 , Remo P. J. Tilanus 17 , Michael Titus 8 , Pablo Torne 9,16 , Jan Wagner 9 , Jonathan Weintroub 1,2 , Maciek Wielgus 9 , André Young 18 , Kazunori Akiyama 1,8,19 , Ezequiel Albentosa-Ruíz 20 , Antxon Alberdi 21 , Walter Alef 9 , Juan Carlos Algaba 22 , Richard Anantua 1,2,23 , Rebecca Azulay 9,20,24 , Uwe Bach 9 , Anne-Kathrin Baczko 9,25 , David Ball 17 , Mislav Balokovic 26 , Bidisha Bandyopadhyay 13 , John Barrett 8 , Michi Bauböck 27 , Bradford A. Benson 28,29 , Dan Bintley 30,31 , Raymond Blundell 2 , Katherine L. Bouman 32 , Hope Boyce 33,34 , Roger Brissenden 1,2 , Silke Britzen 9 , Avery E. Broderick 35,36,37 , Thomas Bronzwaer 18 , Sandra Bustamante 38 , John E. Carlstrom 29,39,40,41 , Andrew Chael 42 , Chi-kwan Chan 17,43,44 , Dominic O. Chang 1,2 , Koushik Chatterjee 1,2 , Shami Chatterjee 45 , Yongjun Chen (陈永军) 46,47 , Xiaopeng Cheng 48 , Ilje Cho 21,48,49 , Pierre Christian 50 , Nicholas S. Conroy 2,51 , John E. Conway 25 , Thomas M. Crawford 29,39 , Alejandro Cruz-Osorio 52,53 , Yuzhu Cui (崔玉竹) 54,55 , Rohan Dahale 21 , Jordy Davelaar 18,56,57 , Mariafelicia De Laurentis 58,59 , Roger Deane 60,61,62 , Jessica Dempsey 30,31,63 , Gregory Desvignes 9,64 , Jason Dexter 65 , Vedant Dhruv 27 , Indu K. Dihingia 55 , Sergio A. Dzib 9,7 , Ralph P. Eatough 9,66 , Razieh Emami 2 , Heino Falcke 18 , Joseph Farah 67,68 , Edward Fomalont 69 , Anne-Laure Fontana 7 , H. Alyson Ford 17 , Marianna Foschi 21 , Raquel Fraga-Encinas 18 , William T. Freeman 70,71 , Per Friberg 30,31 , Christian M. Fromm 9,53,72 , Antonio Fuentes 21 , Peter Galison 1,73,74 , Charles F. Gammie 27,51,75 , Boris Georgiev 17 , Roman Gold 76 , Arturo I. Gómez-Ruiz 77,78 , José L. Gómez 21 , Minfeng Gu (顾敏峰) 46,79 , Mark Gurwell 2 , Kazuhiro Hada 80,81 , Daryl Haggard 33,34 , Ronald Hesper 82 , Dirk Heumann 17 , Luis C. Ho (何子山) 83,84 , Paul Ho 4,30,31 , Mareki Honma 80,81,85 , Chih-Wei L. Huang 4 , Lei Huang (黄磊) 46,79 , David H. Hughes 77 , Shiro Ikeda 19,86,87,88 , C. M. Violette Impellizzeri 69,89 , Makoto Inoue 4 , Sara Issaoun 2,150 , David J. James 90,91 , Buell T. Jannuzi 17 , Britton Jeter 4 , Wu Jiang (江悟) 46 , Alejandra Jiménez-Rosales 18 , Michael D. Johnson 1,2 , Svetlana Jorstad 92 , Adam C. Jones 29 , Abhishek V. Joshi 27 , Taehyun Jung 48,93 , Ramesh Karuppusamy 9 , Tomohisa Kawashima 94 , Mark Kettenis 95 , Dong-Jin Kim 8 , Jae-Young Kim 9,96 , Jongsoo Kim 48 , Junhan Kim 97 , Motoki Kino 19,98 , Prashant Kocherlakota 53 , Yutaro Kofuji 80,85 , Patrick M. Koch 4 , Shoko Koyama 4,99 , Carsten Kramer 7 , Joana A. Kramer 9 , Michael Kramer 9 , Derek Kubo 5 , Cheng-Yu Kuo 4,100 , Noemi La Bella 18 , Sang-Sung Lee 48 , Aviad Levis 32 , Zhiyuan Li (李志远) 101,102 , Rocco Lico 21,103 , Greg Lindahl 2 , Michael Lindqvist 25 , Mikhail Lisakov 9 , Jun Liu (刘俊) 9 , Kuo Liu 9 , Elisabetta Liuzzo 104 , Andrei P. Lobanov 9 , Laurent Loinard 105 , Colin J. Lonsdale 8 , Amy E. Lowitz 17 , Ru-Sen Lu (路如森) 9,46,47 , Nicholas R. MacDonald 9 , Sylvain Mahieu 7 , Doris Maier 7 , Jirong Mao (毛基荣) 106,107,108 , Nicola Marchili 9,104 , Sera Markoff 109,110 , Daniel P. Marrone 17 , Alan P. Marscher 92 , Iván Martí-Vidal 20,24 , Lia Medeiros 111,150 , Karl M. Menten 9 , Izumi Mizuno 30,31 , Yosuke Mizuno 53,55,112 , Joshua Montgomery 29,34 , Kotaro Moriyama 53,80 , Monika Moscibrodzka 18 , Wanga Mulaudzi 109 , Cornelia Müller 9,18 , Hendrik Müller 9 , Alejandro Mus 20,24 , Gibwa Musoke 18,109 , Ioannis Myserlis 16 , Hiroshi Nagai 19,81 , Neil M. Nagar 13 , Masanori Nakamura 4,113 , Gopal Narayanan 38 , Iniyan Natarajan 1,2 , Antonios Nathanail 53,114 , Santiago Navarro Fuentes 16 , Joey Neilsen 115 , Chunchong Ni 35,36,37 , Michael A. Nowak 116 , Junghwan Oh 95 , Hiroki Okino 80,85 , Héctor Raúl Olivares Sánchez 117 , Tomoaki Oyama 80 , Feryal Özel 118 , Daniel C. M. Palumbo 1,2 , Georgios Filippos Paraschos 9 , Jongho Park 119 , Harriet Parsons 30,31 , Ue-Li Pen 4,35,120,121,122 , Vincent Piétu 7 , Aleksandar PopStefanija 38 , Oliver Porth 53,109 , Ben Prather 27 , Giacomo Principe 103,123,124 , Dimitrios Psaltis 118 , Hung-Yi Pu 4,125,126 , Philippe A. Raffin 4 , Ramprasad Rao 2 , Mark G. Rawlings 30,31,127 , Angelo Ricarte 1,2 , Bart Ripperda 35,120,121,128 , Freek Roelofs 1,2,18 , Cristina Romero-Cañizales 4 , Eduardo Ros 9 , Arash Roshanineshat 17 , Ignacio Ruiz 16 , Chet Ruszczyk 8 , Kazi L. J. Rygl 104 , David Sánchez-Argüelles 77,78 , Miguel Sánchez-Portal 16 , Mahito Sasada 80,129,130 , Kaushik Satapathy 17 , Tuomas Savolainen 9,15,131 , F. Peter Schloerb 38 , Jonathan Schonfeld 2 , Karl-Friedrich Schuster 7 , Lijing Shao 9,84 , Zhiqiang Shen (沈志强) 46,47 , Des Small 95 , Bong Won Sohn 48,49,93 , Jason SooHoo 8 , León David Sosapanta Salas 109 , Kamal Souccar 38 , Joshua S. Stanway 132 , He Sun (孙赫) 133,134 , Fumie Tazaki 135 , Alexandra J. Tetarenko 136 , Paul Tiede 1,2 , Kenji Toma 137,138 , Teresa Toscano 21 , Efthalia Traianou 9,21 , Tyler Trent 17 , Sascha Trippe 139 , Matthew Turk 51 , Ilse van Bemmel 95 , Huib Jan van Langevelde 89,95,140 , Daniel R. van Rossum 18 , Jesse Vos 18 , Derek Ward-Thompson 132 , John Wardle 141 , Jasmin E. Washington 17 , Robert Wharton 9 , Kaj Wiik 142 , Gunther Witzel 9 , Michael F. Wondrak 18,143 , George N. Wong 42,144 , Qingwen Wu (吴庆文) 145 , Nitika Yadlapalli 32 , Paul Yamaguchi 2 , Aristomenis Yfantis 18 , Doosoo Yoon 109 , Ziri Younsi 53,146 , Wei Yu (于威) 2 , Feng Yuan (袁峰) 147 , Ye-Fei Yuan (袁业飞) 148 , J. Anton Zensus 9 , Shuo Zhang 149 , Guang-Yao Zhao 9,21 , and Shan-Shan Zhao (赵杉杉) 46
Published 2024 August 27 • © 2024. The Author(s). Published by the American Astronomical Society. The Astronomical Journal , Volume 168 , Number 3 Citation Alexander W. Raymond et al 2024 AJ 168 130 DOI 10.3847/1538-3881/ad5bdb
You need an eReader or compatible software to experience the benefits of the ePub3 file format .
Article metrics
1 Total downloads
Share this article
Author e-mails.
Author affiliations
1 Black Hole Initiative at Harvard University, 20 Garden Street, Cambridge, MA 02138, USA; [email protected]
2 Center for Astrophysics ∣ Harvard & Smithsonian, 60 Garden Street, Cambridge, MA 02138, USA
3 Current address is Jet Propulsion Laboratory, California Institute of Technology, Pasadena, CA, USA
4 Institute of Astronomy and Astrophysics, Academia Sinica, 11F of Astronomy-Mathematics Building, AS/NTU No. 1, Sec. 4, Roosevelt Road, Taipei 106216, Taiwan, R.O.C.
5 Institute of Astronomy and Astrophysics, Academia Sinica, 645 North A'ohoku Place, Hilo, HI 96720, USA
6 Department of Physics and Astronomy, University of Hawaii at Manoa, 2505 Correa Road, Honolulu, HI 96822, USA
7 Institut de Radioastronomie Millimétrique (IRAM), 300 rue de la Piscine, F-38406 Saint Martin d'Hères, France
8 Massachusetts Institute of Technology Haystack Observatory, 99 Millstone Road, Westford, MA 01886, USA
9 Max-Planck-Institut für Radioastronomie, Auf dem Hügel 69, D-53121 Bonn, Germany
10 Dipartimento di Fisica, Universitá degli Studi di Cagliari, SP Monserrato-Sestu km 0.7, I-09042 Monserrato, Italy
11 INAF–Osservatorio Astronomico di Cagliari, Via della Scienza 5, 09047, Selargius, CA, Italy
12 Department of Physics, National Taiwan University, No. 1, Sec. 4, Roosevelt Road, Taipei 106216, Taiwan, R.O.C.
13 Astronomy Department, Universidad de Concepción, Casilla 160-C, Concepción, Chile
14 Finnish Centre for Astronomy with ESO, FI-20014 University of Turku, Finland
15 Aalto University Metsähovi Radio Observatory, Metsähovintie 114, FI-02540 Kylmälä, Finland
16 Institut de Radioastronomie Millimétrique (IRAM), Avenida Divina Pastora 7, Local 20, E-18012, Granada, Spain
17 Steward Observatory and Department of Astronomy, University of Arizona, 933 North Cherry Avenue, Tucson, AZ 85721, USA
18 Department of Astrophysics, Institute for Mathematics, Astrophysics and Particle Physics (IMAPP), Radboud University, P.O. Box 9010, 6500 GL Nijmegen, The Netherlands
19 National Astronomical Observatory of Japan, 2-21-1 Osawa, Mitaka, Tokyo 181-8588, Japan
20 Departament d'Astronomia i Astrofísica, Universitat de València, C. Dr. Moliner 50, E-46100 Burjassot, València, Spain
21 Instituto de Astrofísica de Andalucía-CSIC, Glorieta de la Astronomía s/n, E-18008 Granada, Spain
22 Department of Physics, Faculty of Science, Universiti Malaya, 50603 Kuala Lumpur, Malaysia
23 Department of Physics & Astronomy, The University of Texas at San Antonio, One UTSA Circle, San Antonio, TX 78249, USA
24 Observatori Astronòmic, Universitat de València, C. Catedrático José Beltrán 2, E-46980 Paterna, València, Spain
25 Department of Space, Earth and Environment, Chalmers University of Technology, Onsala Space Observatory, SE-43992 Onsala, Sweden
26 Yale Center for Astronomy & Astrophysics, Yale University, 52 Hillhouse Avenue, New Haven, CT 06511, USA
27 Department of Physics, University of Illinois, 1110 West Green Street, Urbana, IL 61801, USA
28 Fermi National Accelerator Laboratory, MS209, P.O. Box 500, Batavia, IL 60510, USA
29 Department of Astronomy and Astrophysics, University of Chicago, 5640 South Ellis Avenue, Chicago, IL 60637, USA
30 East Asian Observatory, 660 North A'ohoku Place, Hilo, HI 96720, USA
31 James Clerk Maxwell Telescope (JCMT), 660 North A'ohoku Place, Hilo, HI 96720, USA
32 California Institute of Technology, 1200 East California Boulevard, Pasadena, CA 91125, USA
33 Department of Physics, McGill University, 3600 rue University, Montréal, QC H3A 2T8, Canada
34 Trottier Space Institute at McGill, 3550 rue University, Montréal, QC H3A 2A7, Canada
35 Perimeter Institute for Theoretical Physics, 31 Caroline Street North, Waterloo, ON N2L 2Y5, Canada
36 Department of Physics and Astronomy, University of Waterloo, 200 University Avenue West, Waterloo, ON N2L 3G1, Canada
37 Waterloo Centre for Astrophysics, University of Waterloo, Waterloo, ON N2L 3G1, Canada
38 Department of Astronomy, University of Massachusetts, Amherst, MA 01003, USA
39 Kavli Institute for Cosmological Physics, University of Chicago, 5640 South Ellis Avenue, Chicago, IL 60637, USA
40 Department of Physics, University of Chicago, 5720 South Ellis Avenue, Chicago, IL 60637, USA
41 Enrico Fermi Institute, University of Chicago, 5640 South Ellis Avenue, Chicago, IL 60637, USA
42 Princeton Gravity Initiative, Jadwin Hall, Princeton University, Princeton, NJ 08544, USA
43 Data Science Institute, University of Arizona, 1230 North Cherry Avenue, Tucson, AZ 85721, USA
44 Program in Applied Mathematics, University of Arizona, 617 North Santa Rita, Tucson, AZ 85721, USA
45 Cornell Center for Astrophysics and Planetary Science, Cornell University, Ithaca, NY 14853, USA
46 Shanghai Astronomical Observatory, Chinese Academy of Sciences, 80 Nandan Road, Shanghai 200030, People's Republic of China
47 Key Laboratory of Radio Astronomy and Technology, Chinese Academy of Sciences, A20 Datun Road, Chaoyang District, Beijing, 100101, People's Republic of China
48 Korea Astronomy and Space Science Institute, Daedeok-daero 776, Yuseong-gu, Daejeon 34055, Republic of Korea
49 Department of Astronomy, Yonsei University, Yonsei-ro 50, Seodaemun-gu, 03722 Seoul, Republic of Korea
50 Physics Department, Fairfield University, 1073 North Benson Road, Fairfield, CT 06824, USA
51 Department of Astronomy, University of Illinois at Urbana-Champaign, 1002 West Green Street, Urbana, IL 61801, USA
52 Instituto de Astronomía, Universidad Nacional Autónoma de México (UNAM), Apdo Postal 70-264, Ciudad de México, Mexico
53 Institut für Theoretische Physik, Goethe-Universität Frankfurt, Max-von-Laue-Straße 1, D-60438 Frankfurt am Main, Germany
54 Research Center for Astronomical Computing, Zhejiang Laboratory, Hangzhou 311100, People's Republic of China
55 Tsung-Dao Lee Institute, Shanghai Jiao Tong University, Shengrong Road 520, Shanghai, 201210, People's Republic of China
56 Department of Astronomy and Columbia Astrophysics Laboratory, Columbia University, 500 West 120th Street, New York, NY 10027, USA
57 Center for Computational Astrophysics, Flatiron Institute, 162 Fifth Avenue, New York, NY 10010, USA
58 Dipartimento di Fisica "E. Pancini", Università di Napoli "Federico II", Compl. Univ. di Monte S. Angelo, Edificio G, Via Cinthia, I-80126, Napoli, Italy
59 INFN Sez. di Napoli, Compl. Univ. di Monte S. Angelo, Edificio G, Via Cinthia, I-80126, Napoli, Italy
60 Wits Centre for Astrophysics, University of the Witwatersrand, 1 Jan Smuts Avenue, Braamfontein, Johannesburg 2050, South Africa
61 Department of Physics, University of Pretoria, Hatfield, Pretoria 0028, South Africa
62 Centre for Radio Astronomy Techniques and Technologies, Department of Physics and Electronics, Rhodes University, Makhanda 6140, South Africa
63 ASTRON, Oude Hoogeveensedijk 4, 7991 PD Dwingeloo, The Netherlands
64 LESIA, Observatoire de Paris, Université PSL, CNRS, Sorbonne Université, Université de Paris, 5 place Jules Janssen, F-92195 Meudon, France
65 JILA and Department of Astrophysical and Planetary Sciences, University of Colorado, Boulder, CO 80309, USA
66 National Astronomical Observatories, Chinese Academy of Sciences, 20A Datun Road, Chaoyang District, Beijing 100101, People's Republic of China
67 Las Cumbres Observatory, 6740 Cortona Drive, Suite 102, Goleta, CA 93117-5575, USA
68 Department of Physics, University of California, Santa Barbara, CA 93106-9530, USA
69 National Radio Astronomy Observatory, 520 Edgemont Road, Charlottesville, VA 22903, USA
70 Department of Electrical Engineering and Computer Science, Massachusetts Institute of Technology, 32-D476, 77 Massachusetts Avenue, Cambridge, MA 02142, USA
71 Google Research, 355 Main Street, Cambridge, MA 02142, USA
72 Institut für Theoretische Physik und Astrophysik, Universität Würzburg, Emil-Fischer-Str. 31, D-97074 Würzburg, Germany
73 Department of History of Science, Harvard University, Cambridge, MA 02138, USA
74 Department of Physics, Harvard University, Cambridge, MA 02138, USA
75 NCSA, University of Illinois, 1205 West Clark Street, Urbana, IL 61801, USA
76 CP3-Origins, University of Southern Denmark, Campusvej 55, DK-5230 Odense M, Denmark
77 Instituto Nacional de Astrofísica, Óptica y Electrónica. Apartado Postal 51 y 216, 72000. Puebla Pue., Mexico
78 Consejo Nacional de Humanidades, Ciencia y Tecnología, Av. Insurgentes Sur 1582, 03940, Ciudad de México, Mexico
79 Key Laboratory for Research in Galaxies and Cosmology, Chinese Academy of Sciences, Shanghai 200030, People's Republic of China
80 Mizusawa VLBI Observatory, National Astronomical Observatory of Japan, 2-12 Hoshigaoka, Mizusawa, Oshu, Iwate 023-0861, Japan
81 Department of Astronomical Science, The Graduate University for Advanced Studies (SOKENDAI), 2-21-1 Osawa, Mitaka, Tokyo 181-8588, Japan
82 NOVA Sub-mm Instrumentation Group, Kapteyn Astronomical Institute, University of Groningen, Landleven 12, 9747 AD Groningen, The Netherlands
83 Department of Astronomy, School of Physics, Peking University, Beijing 100871, People's Republic of China
84 Kavli Institute for Astronomy and Astrophysics, Peking University, Beijing 100871, People's Republic of China
85 Department of Astronomy, Graduate School of Science, The University of Tokyo, 7-3-1 Hongo, Bunkyo-ku, Tokyo 113-0033, Japan
86 The Institute of Statistical Mathematics, 10-3 Midori-cho, Tachikawa, Tokyo, 190-8562, Japan
87 Department of Statistical Science, The Graduate University for Advanced Studies (SOKENDAI), 10-3 Midori-cho, Tachikawa, Tokyo 190-8562, Japan
88 Kavli Institute for the Physics and Mathematics of the Universe, The University of Tokyo, 5-1-5 Kashiwanoha, Kashiwa, 277-8583, Japan
89 Leiden Observatory, Leiden University, Postbus 2300, 9513 RA Leiden, The Netherlands
90 ASTRAVEO LLC, PO Box 1668, Gloucester, MA 01931, USA
91 Applied Materials Inc., 35 Dory Road, Gloucester, MA 01930, USA
92 Institute for Astrophysical Research, Boston University, 725 Commonwealth Avenue, Boston, MA 02215, USA
93 University of Science and Technology, Gajeong-ro 217, Yuseong-gu, Daejeon 34113, Republic of Korea
94 Institute for Cosmic Ray Research, The University of Tokyo, 5-1-5 Kashiwanoha, Kashiwa, Chiba 277-8582, Japan
95 Joint Institute for VLBI ERIC (JIVE), Oude Hoogeveensedijk 4, 7991 PD Dwingeloo, The Netherlands
96 Department of Astronomy and Atmospheric Sciences, Kyungpook National University, Daegu 702-701, Republic of Korea
97 Department of Physics, Korea Advanced Institute of Science and Technology (KAIST), 291 Daehak-ro, Yuseong-gu, Daejeon 34141, Republic of Korea
98 Kogakuin University of Technology & Engineering, Academic Support Center, 2665-1 Nakano, Hachioji, Tokyo 192-0015, Japan
99 Graduate School of Science and Technology, Niigata University, 8050 Ikarashi 2-no-cho, Nishi-ku, Niigata 950-2181, Japan
100 Physics Department, National Sun Yat-Sen University, No. 70, Lien-Hai Road, Kaosiung City 80424, Taiwan, R.O.C.
101 School of Astronomy and Space Science, Nanjing University, Nanjing 210023, People's Republic of China
102 Key Laboratory of Modern Astronomy and Astrophysics, Nanjing University, Nanjing 210023, People's Republic of China
103 INAF-Istituto di Radioastronomia, Via P. Gobetti 101, I-40129 Bologna, Italy
104 INAF-Istituto di Radioastronomia & Italian ALMA Regional Centre, Via P. Gobetti 101, I-40129 Bologna, Italy
105 Instituto de Radioastronomía y Astrofísica, Universidad Nacional Autónoma de México, Morelia 58089, Mexico
106 Yunnan Observatories, Chinese Academy of Sciences, 650011 Kunming, Yunnan Province, People's Republic of China
107 Center for Astronomical Mega-Science, Chinese Academy of Sciences, 20A Datun Road, Chaoyang District, Beijing, 100012, People's Republic of China
108 Key Laboratory for the Structure and Evolution of Celestial Objects, Chinese Academy of Sciences, 650011 Kunming, People's Republic of China
109 Anton Pannekoek Institute for Astronomy, University of Amsterdam, Science Park 904, 1098 XH, Amsterdam, The Netherlands
110 Gravitation and Astroparticle Physics Amsterdam (GRAPPA) Institute, University of Amsterdam, Science Park 904, 1098 XH Amsterdam, The Netherlands
111 Department of Astrophysical Sciences, Peyton Hall, Princeton University, Princeton, NJ 08544, USA
112 School of Physics and Astronomy, Shanghai Jiao Tong University, 800 Dongchuan Road, Shanghai, 200240, People's Republic of China
113 National Institute of Technology, Hachinohe College, 16-1 Uwanotai, Tamonoki, Hachinohe City, Aomori 039-1192, Japan
114 Research Center for Astronomy, Academy of Athens, Soranou Efessiou 4, 115 27 Athens, Greece
115 Department of Physics, Villanova University, 800 Lancaster Avenue, Villanova, PA 19085, USA
116 Physics Department, Washington University, CB 1105, St. Louis, MO 63130, USA
117 Departamento de Matemática da Universidade de Aveiro and Centre for Research and Development in Mathematics and Applications (CIDMA), Campus de Santiago, 3810-193 Aveiro, Portugal
118 School of Physics, Georgia Institute of Technology, 837 State Street NW, Atlanta, GA 30332, USA
119 School of Space Research, Kyung Hee University, 1732, Deogyeong-daero, Giheung-gu, Yongin-si, Gyeonggi-do 17104, Republic of Korea
120 Canadian Institute for Theoretical Astrophysics, University of Toronto, 60 St. George Street, Toronto, ON M5S 3H8, Canada
121 Dunlap Institute for Astronomy and Astrophysics, University of Toronto, 50 St. George Street, Toronto, ON M5S 3H4, Canada
122 Canadian Institute for Advanced Research, 180 Dundas Street West, Toronto, ON M5G 1Z8, Canada
123 Dipartimento di Fisica, Università di Trieste, I-34127 Trieste, Italy
124 INFN Sez. di Trieste, I-34127 Trieste, Italy
125 Department of Physics, National Taiwan Normal University, No. 88, Sec. 4, Tingzhou Road, Taipei 116, Taiwan, R.O.C.
126 Center of Astronomy and Gravitation, National Taiwan Normal University, No. 88, Sec. 4, Tingzhou Road, Taipei 116, Taiwan, R.O.C.
127 Gemini Observatory/NSF's NOIRLab, 670 North A'ohoku Place, Hilo, HI 96720, USA
128 Department of Physics, University of Toronto, 60 St. George Street, Toronto, ON M5S 1A7, Canada
129 Department of Physics, Tokyo Institute of Technology, 2-12-1 Ookayama, Meguro-ku, Tokyo 152-8551, Japan
130 Hiroshima Astrophysical Science Center, Hiroshima University, 1-3-1 Kagamiyama, Higashi-Hiroshima, Hiroshima 739-8526, Japan
131 Aalto University Department of Electronics and Nanoengineering, PL 15500, FI-00076 Aalto, Finland
132 Jeremiah Horrocks Institute, University of Central Lancashire, Preston PR1 2HE, UK
133 National Biomedical Imaging Center, Peking University, Beijing 100871, People's Republic of China
134 College of Future Technology, Peking University, Beijing 100871, People's Republic of China
135 Tokyo Electron Technology Solutions Limited, 52 Matsunagane, Iwayado, Esashi, Oshu, Iwate 023-1101, Japan
136 Department of Physics and Astronomy, University of Lethbridge, Lethbridge, AB T1K 3M4, Canada
137 Frontier Research Institute for Interdisciplinary Sciences, Tohoku University, Sendai 980-8578, Japan
138 Astronomical Institute, Tohoku University, Sendai 980-8578, Japan
139 Department of Physics and Astronomy, Seoul National University, Gwanak-gu, Seoul 08826, Republic of Korea
140 University of New Mexico, Department of Physics and Astronomy, Albuquerque, NM 87131, USA
141 Physics Department, Brandeis University, 415 South Street, Waltham, MA 02453, USA
142 Tuorla Observatory, Department of Physics and Astronomy, University of Turku, Finland
143 Radboud Excellence Fellow of Radboud University, Nijmegen, The Netherlands
144 School of Natural Sciences, Institute for Advanced Study, 1 Einstein Drive, Princeton, NJ 08540, USA
145 School of Physics, Huazhong University of Science and Technology, Wuhan, Hubei, 430074, People's Republic of China
146 Mullard Space Science Laboratory, University College London, Holmbury St. Mary, Dorking, Surrey, RH5 6NT, UK
147 Center for Astronomy and Astrophysics and Department of Physics, Fudan University, Shanghai 200438, People's Republic of China
148 Astronomy Department, University of Science and Technology of China, Hefei 230026, People's Republic of China
149 Department of Physics and Astronomy, Michigan State University, 567 Wilson Road, East Lansing, MI 48824, USA
Author notes
150 NASA Hubble Fellowship Program, Einstein Fellow.
151 Corresponding author.
Alexander W. Raymond https://orcid.org/0000-0002-5779-4767
Sheperd S. Doeleman https://orcid.org/0000-0002-9031-0904
Keiichi Asada https://orcid.org/0000-0001-6988-8763
Lindy Blackburn https://orcid.org/0000-0002-9030-642X
Geoffrey C. Bower https://orcid.org/0000-0003-4056-9982
Michael Bremer https://orcid.org/0000-0001-7511-3745
Dominique Broguiere https://orcid.org/0000-0001-9151-6683
Ming-Tang Chen https://orcid.org/0000-0001-6573-3318
Geoffrey B. Crew https://orcid.org/0000-0002-2079-3189
Sven Dornbusch https://orcid.org/0000-0003-3211-3352
Vincent L. Fish https://orcid.org/0000-0002-7128-9345
Roberto García https://orcid.org/0000-0002-6584-7443
Olivier Gentaz https://orcid.org/0000-0002-0115-4605
Ciriaco Goddi https://orcid.org/0000-0002-2542-7743
Michael H. Hecht https://orcid.org/0000-0002-4114-4583
Yau-De Huang https://orcid.org/0000-0001-8783-6211
Michael Janssen https://orcid.org/0000-0001-8685-6544
Garrett K. Keating https://orcid.org/0000-0002-3490-146X
Jun Yi Koay https://orcid.org/0000-0002-7029-6658
Thomas P. Krichbaum https://orcid.org/0000-0002-4892-9586
Wen-Ping Lo https://orcid.org/0000-0003-1869-2503
Satoki Matsushita https://orcid.org/0000-0002-2127-7880
Lynn D. Matthews https://orcid.org/0000-0002-3728-8082
James M. Moran https://orcid.org/0000-0002-3882-4414
Nimesh Patel https://orcid.org/0000-0002-6021-9421
Dominic W. Pesce https://orcid.org/0000-0002-5278-9221
Venkatessh Ramakrishnan https://orcid.org/0000-0002-9248-086X
Alan L. Roy https://orcid.org/0000-0002-1931-0135
Salvador Sánchez https://orcid.org/0000-0002-8042-5951
Remo P. J. Tilanus https://orcid.org/0000-0002-6514-553X
Michael Titus https://orcid.org/0000-0001-9001-3275
Pablo Torne https://orcid.org/0000-0001-8700-6058
Jan Wagner https://orcid.org/0000-0003-1105-6109
Jonathan Weintroub https://orcid.org/0000-0002-4603-5204
Maciek Wielgus https://orcid.org/0000-0002-8635-4242
André Young https://orcid.org/0000-0003-0000-2682
Kazunori Akiyama https://orcid.org/0000-0002-9475-4254
Ezequiel Albentosa-Ruíz https://orcid.org/0000-0002-7816-6401
Antxon Alberdi https://orcid.org/0000-0002-9371-1033
Juan Carlos Algaba https://orcid.org/0000-0001-6993-1696
Richard Anantua https://orcid.org/0000-0003-3457-7660
Rebecca Azulay https://orcid.org/0000-0002-2200-5393
Uwe Bach https://orcid.org/0000-0002-7722-8412
Anne-Kathrin Baczko https://orcid.org/0000-0003-3090-3975
Mislav Balokovic https://orcid.org/0000-0003-0476-6647
Bidisha Bandyopadhyay https://orcid.org/0000-0002-2138-8564
John Barrett https://orcid.org/0000-0002-9290-0764
Michi Bauböck https://orcid.org/0000-0002-5518-2812
Bradford A. Benson https://orcid.org/0000-0002-5108-6823
Raymond Blundell https://orcid.org/0000-0002-5929-5857
Katherine L. Bouman https://orcid.org/0000-0003-0077-4367
Hope Boyce https://orcid.org/0000-0002-6530-5783
Roger Brissenden https://orcid.org/0000-0002-2556-0894
Silke Britzen https://orcid.org/0000-0001-9240-6734
Avery E. Broderick https://orcid.org/0000-0002-3351-760X
Thomas Bronzwaer https://orcid.org/0000-0003-1151-3971
Sandra Bustamante https://orcid.org/0000-0001-6169-1894
John E. Carlstrom https://orcid.org/0000-0002-2044-7665
Andrew Chael https://orcid.org/0000-0003-2966-6220
Chi-kwan Chan https://orcid.org/0000-0001-6337-6126
Dominic O. Chang https://orcid.org/0000-0001-9939-5257
Koushik Chatterjee https://orcid.org/0000-0002-2825-3590
Shami Chatterjee https://orcid.org/0000-0002-2878-1502
Yongjun Chen https://orcid.org/0000-0001-5650-6770
Xiaopeng Cheng https://orcid.org/0000-0003-4407-9868
Ilje Cho https://orcid.org/0000-0001-6083-7521
Pierre Christian https://orcid.org/0000-0001-6820-9941
Nicholas S. Conroy https://orcid.org/0000-0003-2886-2377
John E. Conway https://orcid.org/0000-0003-2448-9181
Thomas M. Crawford https://orcid.org/0000-0001-9000-5013
Alejandro Cruz-Osorio https://orcid.org/0000-0002-3945-6342
Yuzhu Cui https://orcid.org/0000-0001-6311-4345
Rohan Dahale https://orcid.org/0000-0001-6982-9034
Jordy Davelaar https://orcid.org/0000-0002-2685-2434
Mariafelicia De Laurentis https://orcid.org/0000-0002-9945-682X
Roger Deane https://orcid.org/0000-0003-1027-5043
Jessica Dempsey https://orcid.org/0000-0003-1269-9667
Gregory Desvignes https://orcid.org/0000-0003-3922-4055
Jason Dexter https://orcid.org/0000-0003-3903-0373
Vedant Dhruv https://orcid.org/0000-0001-6765-877X
Indu K. Dihingia https://orcid.org/0000-0002-4064-0446
Sergio A. Dzib https://orcid.org/0000-0001-6010-6200
Ralph P. Eatough https://orcid.org/0000-0001-6196-4135
Razieh Emami https://orcid.org/0000-0002-2791-5011
Heino Falcke https://orcid.org/0000-0002-2526-6724
Joseph Farah https://orcid.org/0000-0003-4914-5625
Edward Fomalont https://orcid.org/0000-0002-9036-2747
H. Alyson Ford https://orcid.org/0000-0002-9797-0972
Marianna Foschi https://orcid.org/0000-0001-8147-4993
Raquel Fraga-Encinas https://orcid.org/0000-0002-5222-1361
Per Friberg https://orcid.org/0000-0002-8010-8454
Christian M. Fromm https://orcid.org/0000-0002-1827-1656
Antonio Fuentes https://orcid.org/0000-0002-8773-4933
Peter Galison https://orcid.org/0000-0002-6429-3872
Charles F. Gammie https://orcid.org/0000-0001-7451-8935
Boris Georgiev https://orcid.org/0000-0002-3586-6424
Roman Gold https://orcid.org/0000-0003-2492-1966
Arturo I. Gómez-Ruiz https://orcid.org/0000-0001-9395-1670
José L. Gómez https://orcid.org/0000-0003-4190-7613
Minfeng Gu https://orcid.org/0000-0002-4455-6946
Mark Gurwell https://orcid.org/0000-0003-0685-3621
Kazuhiro Hada https://orcid.org/0000-0001-6906-772X
Daryl Haggard https://orcid.org/0000-0001-6803-2138
Ronald Hesper https://orcid.org/0000-0003-1918-6098
Dirk Heumann https://orcid.org/0000-0002-7671-0047
Luis C. Ho https://orcid.org/0000-0001-6947-5846
Paul Ho https://orcid.org/0000-0002-3412-4306
Mareki Honma https://orcid.org/0000-0003-4058-9000
Chih-Wei L. Huang https://orcid.org/0000-0001-5641-3953
Lei Huang https://orcid.org/0000-0002-1923-227X
Shiro Ikeda https://orcid.org/0000-0002-2462-1448
C. M. Violette Impellizzeri https://orcid.org/0000-0002-3443-2472
Makoto Inoue https://orcid.org/0000-0001-5037-3989
Sara Issaoun https://orcid.org/0000-0002-5297-921X
David J. James https://orcid.org/0000-0001-5160-4486
Buell T. Jannuzi https://orcid.org/0000-0002-1578-6582
Britton Jeter https://orcid.org/0000-0003-2847-1712
Wu Jiang https://orcid.org/0000-0001-7369-3539
Alejandra Jiménez-Rosales https://orcid.org/0000-0002-2662-3754
Michael D. Johnson https://orcid.org/0000-0002-4120-3029
Svetlana Jorstad https://orcid.org/0000-0001-6158-1708
Abhishek V. Joshi https://orcid.org/0000-0002-2514-5965
Taehyun Jung https://orcid.org/0000-0001-7003-8643
Ramesh Karuppusamy https://orcid.org/0000-0002-5307-2919
Tomohisa Kawashima https://orcid.org/0000-0001-8527-0496
Mark Kettenis https://orcid.org/0000-0002-6156-5617
Dong-Jin Kim https://orcid.org/0000-0002-7038-2118
Jae-Young Kim https://orcid.org/0000-0001-8229-7183
Jongsoo Kim https://orcid.org/0000-0002-1229-0426
Junhan Kim https://orcid.org/0000-0002-4274-9373
Motoki Kino https://orcid.org/0000-0002-2709-7338
Prashant Kocherlakota https://orcid.org/0000-0001-7386-7439
Patrick M. Koch https://orcid.org/0000-0003-2777-5861
Shoko Koyama https://orcid.org/0000-0002-3723-3372
Carsten Kramer https://orcid.org/0000-0002-4908-4925
Joana A. Kramer https://orcid.org/0009-0003-3011-0454
Michael Kramer https://orcid.org/0000-0002-4175-2271
Cheng-Yu Kuo https://orcid.org/0000-0001-6211-5581
Noemi La Bella https://orcid.org/0000-0002-8116-9427
Sang-Sung Lee https://orcid.org/0000-0002-6269-594X
Aviad Levis https://orcid.org/0000-0001-7307-632X
Zhiyuan Li https://orcid.org/0000-0003-0355-6437
Rocco Lico https://orcid.org/0000-0001-7361-2460
Greg Lindahl https://orcid.org/0000-0002-6100-4772
Michael Lindqvist https://orcid.org/0000-0002-3669-0715
Mikhail Lisakov https://orcid.org/0000-0001-6088-3819
Jun Liu https://orcid.org/0000-0002-7615-7499
Kuo Liu https://orcid.org/0000-0002-2953-7376
Elisabetta Liuzzo https://orcid.org/0000-0003-0995-5201
Andrei P. Lobanov https://orcid.org/0000-0003-1622-1484
Laurent Loinard https://orcid.org/0000-0002-5635-3345
Colin J. Lonsdale https://orcid.org/0000-0003-4062-4654
Amy E. Lowitz https://orcid.org/0000-0002-4747-4276
Ru-Sen Lu https://orcid.org/0000-0002-7692-7967
Nicholas R. MacDonald https://orcid.org/0000-0002-6684-8691
Jirong Mao https://orcid.org/0000-0002-7077-7195
Nicola Marchili https://orcid.org/0000-0002-5523-7588
Sera Markoff https://orcid.org/0000-0001-9564-0876
Daniel P. Marrone https://orcid.org/0000-0002-2367-1080
Alan P. Marscher https://orcid.org/0000-0001-7396-3332
Iván Martí-Vidal https://orcid.org/0000-0003-3708-9611
Lia Medeiros https://orcid.org/0000-0003-2342-6728
Karl M. Menten https://orcid.org/0000-0001-6459-0669
Izumi Mizuno https://orcid.org/0000-0002-7210-6264
Yosuke Mizuno https://orcid.org/0000-0002-8131-6730
Joshua Montgomery https://orcid.org/0000-0003-0345-8386
Kotaro Moriyama https://orcid.org/0000-0003-1364-3761
Monika Moscibrodzka https://orcid.org/0000-0002-4661-6332
Wanga Mulaudzi https://orcid.org/0000-0003-4514-625X
Cornelia Müller https://orcid.org/0000-0002-2739-2994
Hendrik Müller https://orcid.org/0000-0002-9250-0197
Alejandro Mus https://orcid.org/0000-0003-0329-6874
Gibwa Musoke https://orcid.org/0000-0003-1984-189X
Ioannis Myserlis https://orcid.org/0000-0003-3025-9497
Hiroshi Nagai https://orcid.org/0000-0003-0292-3645
Neil M. Nagar https://orcid.org/0000-0001-6920-662X
Masanori Nakamura https://orcid.org/0000-0001-6081-2420
Gopal Narayanan https://orcid.org/0000-0002-4723-6569
Iniyan Natarajan https://orcid.org/0000-0001-8242-4373
Antonios Nathanail https://orcid.org/0000-0002-1655-9912
Joey Neilsen https://orcid.org/0000-0002-8247-786X
Chunchong Ni https://orcid.org/0000-0003-1361-5699
Michael A. Nowak https://orcid.org/0000-0001-6923-1315
Junghwan Oh https://orcid.org/0000-0002-4991-9638
Hiroki Okino https://orcid.org/0000-0003-3779-2016
Héctor Raúl Olivares Sánchez https://orcid.org/0000-0001-6833-7580
Tomoaki Oyama https://orcid.org/0000-0003-4046-2923
Feryal Özel https://orcid.org/0000-0003-4413-1523
Daniel C. M. Palumbo https://orcid.org/0000-0002-7179-3816
Georgios Filippos Paraschos https://orcid.org/0000-0001-6757-3098
Jongho Park https://orcid.org/0000-0001-6558-9053
Harriet Parsons https://orcid.org/0000-0002-6327-3423
Ue-Li Pen https://orcid.org/0000-0003-2155-9578
Vincent Piétu https://orcid.org/0009-0006-3497-397X
Oliver Porth https://orcid.org/0000-0002-4584-2557
Ben Prather https://orcid.org/0000-0002-0393-7734
Giacomo Principe https://orcid.org/0000-0003-0406-7387
Dimitrios Psaltis https://orcid.org/0000-0003-1035-3240
Hung-Yi Pu https://orcid.org/0000-0001-9270-8812
Ramprasad Rao https://orcid.org/0000-0002-1407-7944
Mark G. Rawlings https://orcid.org/0000-0002-6529-202X
Angelo Ricarte https://orcid.org/0000-0001-5287-0452
Bart Ripperda https://orcid.org/0000-0002-7301-3908
Freek Roelofs https://orcid.org/0000-0001-5461-3687
Cristina Romero-Cañizales https://orcid.org/0000-0001-6301-9073
Eduardo Ros https://orcid.org/0000-0001-9503-4892
Arash Roshanineshat https://orcid.org/0000-0002-8280-9238
Ignacio Ruiz https://orcid.org/0000-0002-0965-5463
Chet Ruszczyk https://orcid.org/0000-0001-7278-9707
Kazi L. J. Rygl https://orcid.org/0000-0003-4146-9043
David Sánchez-Argüelles https://orcid.org/0000-0002-7344-9920
Miguel Sánchez-Portal https://orcid.org/0000-0003-0981-9664
Mahito Sasada https://orcid.org/0000-0001-5946-9960
Kaushik Satapathy https://orcid.org/0000-0003-0433-3585
Tuomas Savolainen https://orcid.org/0000-0001-6214-1085
Jonathan Schonfeld https://orcid.org/0000-0002-8909-2401
Karl-Friedrich Schuster https://orcid.org/0000-0003-2890-9454
Lijing Shao https://orcid.org/0000-0002-1334-8853
Zhiqiang Shen https://orcid.org/0000-0003-3540-8746
Des Small https://orcid.org/0000-0003-3723-5404
Bong Won Sohn https://orcid.org/0000-0002-4148-8378
Jason SooHoo https://orcid.org/0000-0003-1938-0720
León David Sosapanta Salas https://orcid.org/0000-0003-1979-6363
Kamal Souccar https://orcid.org/0000-0001-7915-5272
Joshua S. Stanway https://orcid.org/0009-0003-7659-4642
He Sun https://orcid.org/0000-0003-1526-6787
Fumie Tazaki https://orcid.org/0000-0003-0236-0600
Alexandra J. Tetarenko https://orcid.org/0000-0003-3906-4354
Paul Tiede https://orcid.org/0000-0003-3826-5648
Kenji Toma https://orcid.org/0000-0002-7114-6010
Teresa Toscano https://orcid.org/0000-0003-3658-7862
Efthalia Traianou https://orcid.org/0000-0002-1209-6500
Sascha Trippe https://orcid.org/0000-0003-0465-1559
Matthew Turk https://orcid.org/0000-0002-5294-0198
Ilse van Bemmel https://orcid.org/0000-0001-5473-2950
Huib Jan van Langevelde https://orcid.org/0000-0002-0230-5946
Daniel R. van Rossum https://orcid.org/0000-0001-7772-6131
Jesse Vos https://orcid.org/0000-0003-3349-7394
Derek Ward-Thompson https://orcid.org/0000-0003-1140-2761
John Wardle https://orcid.org/0000-0002-8960-2942
Jasmin E. Washington https://orcid.org/0000-0002-7046-0470
Robert Wharton https://orcid.org/0000-0002-7416-5209
Kaj Wiik https://orcid.org/0000-0002-0862-3398
Gunther Witzel https://orcid.org/0000-0003-2618-797X
Michael F. Wondrak https://orcid.org/0000-0002-6894-1072
George N. Wong https://orcid.org/0000-0001-6952-2147
Qingwen Wu https://orcid.org/0000-0003-4773-4987
Nitika Yadlapalli https://orcid.org/0000-0003-3255-4617
Paul Yamaguchi https://orcid.org/0000-0002-6017-8199
Aristomenis Yfantis https://orcid.org/0000-0002-3244-7072
Doosoo Yoon https://orcid.org/0000-0001-8694-8166
Ziri Younsi https://orcid.org/0000-0001-9283-1191
Wei Yu https://orcid.org/0000-0002-5168-6052
Feng Yuan https://orcid.org/0000-0003-3564-6437
Ye-Fei Yuan https://orcid.org/0000-0002-7330-4756
J. Anton Zensus https://orcid.org/0000-0001-7470-3321
Shuo Zhang https://orcid.org/0000-0002-2967-790X
Guang-Yao Zhao https://orcid.org/0000-0002-4417-1659
Shan-Shan Zhao https://orcid.org/0000-0002-9774-3606
- Received 2024 April 30
- Revised 2024 June 23
- Accepted 2024 June 24
- Published 2024 August 27
Very long baseline interferometry ; Radio interferometry ; Black holes ; Supermassive black holes ; High angular resolution ; Astronomical techniques ; Event horizons
Receive alerts on all new research papers in American Astronomical Society ( A A S ) journals as soon as they are published. Select your desired journals and corridors below. You will need to select a minimum of one corridor.
Please note, The Planetary Science Journal (PSJ) does not currently use the corridors.
What are corridors? opens in new tab
The first very long baseline interferometry (VLBI) detections at 870 μ m wavelength (345 GHz frequency) are reported, achieving the highest diffraction-limited angular resolution yet obtained from the surface of the Earth and the highest-frequency example of the VLBI technique to date. These include strong detections for multiple sources observed on intercontinental baselines between telescopes in Chile, Hawaii, and Spain, obtained during observations in 2018 October. The longest-baseline detections approach 11 G λ , corresponding to an angular resolution, or fringe spacing, of 19 μ as. The Allan deviation of the visibility phase at 870 μ m is comparable to that at 1.3 mm on the relevant integration timescales between 2 and 100 s. The detections confirm that the sensitivity and signal chain stability of stations in the Event Horizon Telescope (EHT) array are suitable for VLBI observations at 870 μ m. Operation at this short wavelength, combined with anticipated enhancements of the EHT, will lead to a unique high angular resolution instrument for black hole studies, capable of resolving the event horizons of supermassive black holes in both space and time.
Export citation and abstract BibTeX RIS
Original content from this work may be used under the terms of the Creative Commons Attribution 4.0 licence . Any further distribution of this work must maintain attribution to the author(s) and the title of the work, journal citation and DOI.
1. Introduction
The technique of very long baseline interferometry (VLBI) involves a network of independently clocked telescopes separated by large distances, which simultaneously observe a common astronomical source (Thompson et al. 2017 ). The angular resolution, or fringe spacing, in a VLBI observation scales inversely with both the distance between stations (i.e., the length of the baseline) and the observing frequency. The present article reports the first fringe detections made at 870 μ m wavelength (345 GHz nominal frequency), which constitutes the shortest-wavelength VLBI observation to date. The experiment we describe was intended as a first technical demonstration of the 870 μ m VLBI capability using facilities that are part of the Event Horizon Telescope (EHT) array. Figure 1 shows the stations that participated in the fringe test along with the usual metric used to characterize millimeter-wavelength observing conditions: the 225 GHz zenith opacity (Thompson et al. 2017 ).
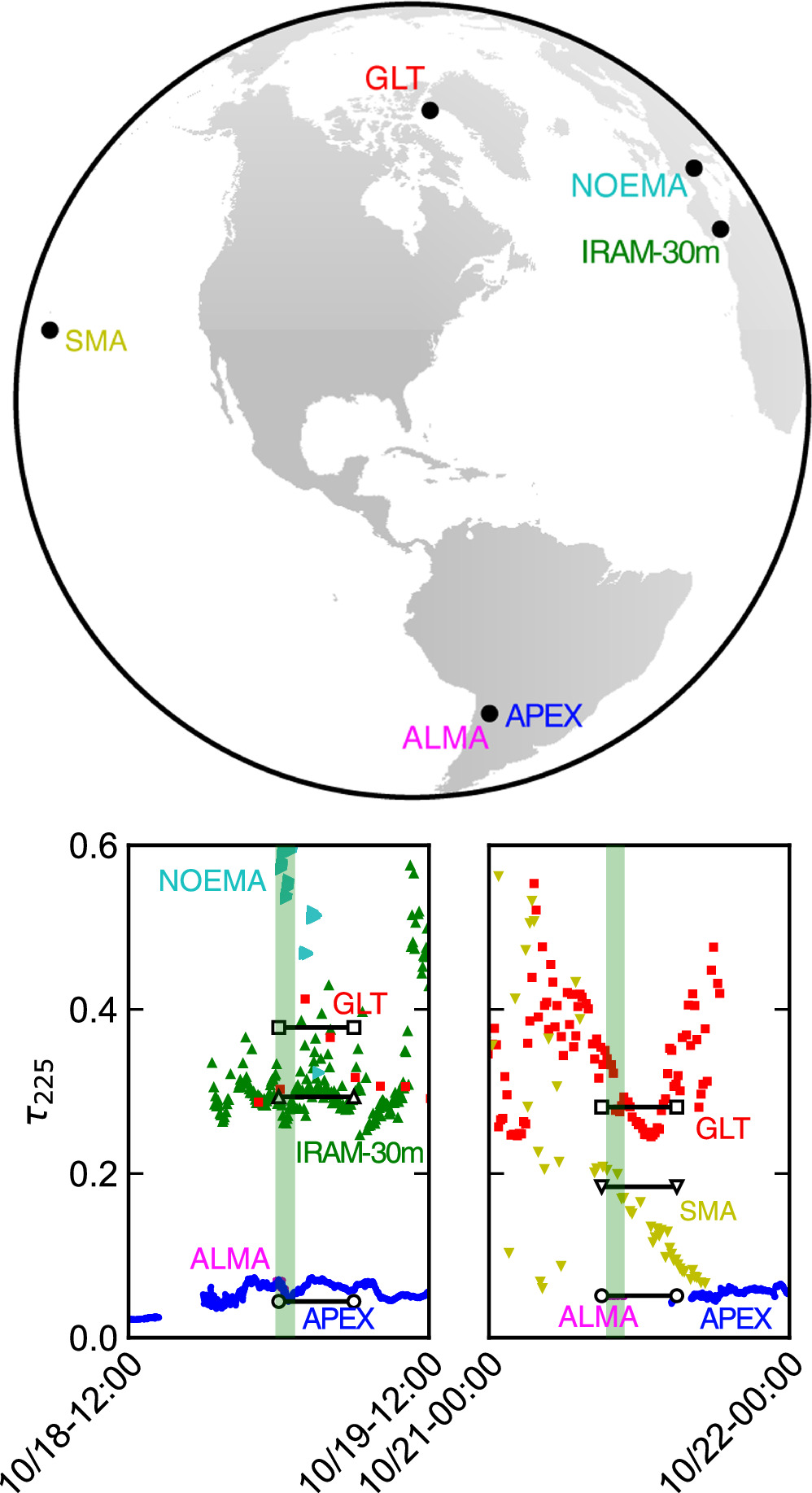
Figure 1. (Top) Stations in the 870 μ m fringe test. (Bottom) Zenith opacity at 225 GHz, which is the standard frequency used for monitoring millimeter-wave conditions. The observing window on each day is indicated by the green shading. Conditions at ALMA were very good during both days ( τ 225 ≈ 0.05). The black lines indicate the opacity at each site calculated using inputs from MERRA-2 reanalysis during the observing windows, which we use to estimate 870 μ m (345 GHz) opacity. Opacities for APEX and NOEMA have been estimated by converting precipitable water vapor column amounts.
Download figure:
The VLBI observing wavelength has decreased over time. The first 3 mm VLBI detections (at 86 GHz) were obtained through observations performed in 1981 (Readhead et al. 1983 ), the first 3 mm intercontinental detections (100 GHz) were obtained through observations performed in 1988 (Baath et al. 1991 , 1992 ), and the first successful 1.3 mm (230 GHz) VLBI was carried out in 1989 (Padin et al. 1990 ). The especially long time since the last significant decrease in VLBI wavelength reflects the challenges of carrying out such observations, which are detailed below. Even so, there have been several milestones of note since the early 1990s on the path toward developing short-wavelength VLBI as an important technique for astrophysics. Increased sensitivity through the use of larger telescopes and advanced receivers led to 1.4 mm (215 GHz) detections on a ∼1100 km baseline of multiple active galactic nuclei (AGN) and Sagittarius A* (Sgr A*), the Galactic center supermassive black hole (Greve et al. 1995 ; Krichbaum et al. 1997 , 1998 ). A return to the longer-wavelength 2 mm spectral windows (147 GHz and 129 GHz) allowed extension of millimeter-wavelength VLBI to intercontinental baselines (Doeleman et al. 2002 ; Greve et al. 2002 ; Krichbaum et al. 2002 ). Building on this work, Doeleman et al. ( 2008 , 2012 ) used purpose-built wideband digital VLBI systems on 1.3 mm transoceanic baselines to report the discovery of event-horizon-scale structures in Sgr A* and the much more massive black hole, M87*. The EHT collaboration has now imaged both of these sources with a global 1.3 mm VLBI array (Event Horizon Telescope Collaboration et al. 2019a , 2022a , 2024 ).
The EHT is the highest-resolution ground-based VLBI instrument to date (Event Horizon Telescope Collaboration et al. 2019b ). The EHT fringe spacing is approximately 25 μ as at 1.3 mm wavelength. The finite diameter of the Earth limits ground-based 1.3 mm fringe spacing to 21 μ as, corresponding to a 9.8 G λ baseline. In practice, modern imaging methods, such as regularized maximum likelihood, achieve a slightly higher angular resolution that exceeds the diffraction limit (Event Horizon Telescope Collaboration et al. 2019c ).
For future campaigns, the EHT has developed the capability to observe at 870 μ m, and enhancing the ability to observe at this wavelength through new stations and wider bandwidth is an important aspect of long-term enhancements envisaged by the next-generation EHT (ngEHT) project (Doeleman et al. 2019 , 2023 ; Raymond et al. 2021 ). For a given set of station locations, observing at 870 μ m improves angular resolution by approximately 50% compared to observing at 1.3 mm, which will provide a sharper view of the black hole shadow and environment; the 870 μ m fringe spacing limit set by the diameter of the Earth is approximately 14 μ as, corresponding to a 14.7 G λ baseline. Observations at 870 μ m are also important for polarimetric measurements. Faraday rotation, which scrambles the imaged electric field vector position angle pattern, diminishes with the square of the frequency. Therefore, 870 μ m observations may help distinguish Faraday rotation from the intrinsic field pattern set by the horizon-scale magnetic field and plasma properties (Event Horizon Telescope Collaboration et al. 2021 ; Wielgus et al. 2024 ). For Sgr A*, the angular size of the black hole shadow is larger than that of M87* (Event Horizon Telescope Collaboration et al. 2022a ), but scattering in the ionized interstellar medium affects the image angular resolution (see, e.g., Johnson et al. 2018 ). At 1.3 mm, the scatter broadening is comparable to the current EHT resolution, but it decreases approximately as the observing wavelength squared. Thus, at 870 μ m, scattering effects would be significantly diminished and would not limit the resolution of a VLBI array for studies of Sgr A*. In particular, extension of the EHT to 870 μ m wavelengths can target photon ring substructure in Sgr A*, aiming to detect the orbit of light that makes a full "u-turn" around the black hole (Johnson et al. 2020 ; Palumbo et al. 2023 ). For these reasons, 870 μ m VLBI opens important new directions for advanced horizon-resolved studies of the two primary EHT sources. At the same time, higher-frequency VLBI brings more sources into range for horizon-resolved black hole studies (Pesce et al. 2021 ; Lo et al. 2023 ; Ramakrishnan et al. 2023 ), and the increased resolution at 870 μ m benefits nonhorizon VLBI studies of AGN jets (e.g., Kim et al. 2020 ; Janssen et al. 2021 ; Issaoun et al. 2022 ; Jorstad et al. 2023 ; Paraschos et al. 2024 ). Additionally, due to reduced opacity, shorter wavelengths probe more compact regions of jetted AGN sources (an example being the core-shift effect; Lobanov 1998 ; Hada et al. 2011 ). Hence, 870 μ m VLBI has the potential to image the jet launching region closer to the central black hole, enabling investigations of the physics behind jet formation, collimation, and acceleration. In particular, the poorly understood limb brightening in transversely resolved inner jets (e.g., Janssen et al. 2021 ) can be studied in much greater detail.
Extension of observing to 870 μ m similarly enhances the capability of the EHT to capture dynamics near the event horizon. In the case of Sgr A*, the dynamical timescale is ∼200 s (10 GM / c 3 ). Simultaneous 1.3 mm and 870 μ m observing can sample sufficient Fourier spatial frequencies within this integration time to allow snapshot imaging using the technique of multifrequency synthesis (MFS; Chael et al. 2023 ). Combining such snapshots will enable recovery of accretion and jet launching kinematics. For M87*, the dynamical timescale is ∼3 days, and data obtained in both 1.3 mm and 870 μ m on sequential days can be combined to form high-fidelity MFS images for time-lapse movie reconstruction of the event horizon environment. Realizing the full scientific potential of 870 μ m VLBI (Johnson et al. 2023 ) will require the planned ngEHT upgrade (Doeleman et al. 2023 ).
While there are clearly many motivating reasons for 870 μ m VLBI observing, a number of factors make the measurements difficult in this short-wavelength regime. The atmosphere is more opaque at 870 μ m than at 1.3 mm (see, for example, Liebe 1985 ; Matsushita et al. 1999 , 2016 , 2022 ), which means that sources are more attenuated and noise levels due to atmospheric emission are elevated. Overall, the effective system temperatures of coherent radio receivers are intrinsically greater at 870 μ m than at 1.3 mm. 152 The aperture efficiency of the collecting optics tends to diminish at high frequency, and the source flux density tends to decrease. In addition, coherence losses due to the VLBI frequency standards used at each site increase with observing frequency (Doeleman et al. 2011 ). The EHT array, conceived as a common, international effort of independent observatories working in the short-millimeter range, has directly addressed these challenges and provides key enabling infrastructure for extension of VLBI to higher frequencies (Event Horizon Telescope Collaboration et al. 2019b ).
The telescopes comprising the EHT array are precision structures sited at high-altitude, low-opacity locations (see, e.g., Levy et al. 1996 ; Mangum et al. 2006 ; Greve & Bremer 2010 ; Chen et al. 2023 and references therein on the design and qualification of such instruments). State-of-the-art instrumentation underpinning the operation of these telescopes, as single-dish facilities and for VLBI, includes cryogenic receivers and wideband digital backends—all refined over many years to optimize performance at millimeter and submillimeter wavelengths. Steady improvements in superconductor–insulator–superconductor junctions have formed the basis for increased bandwidth and sensitivity of millimeter and submillimeter receivers, leading to state-of-the-art systems in use at EHT sites (see Maier et al. 2005 , 2012 ; Tong et al. 2005 , 2013 ; Chenu et al. 2007 , 2016 ; Carter et al. 2012 ; Mahieu et al. 2012 ; Kerr et al. 2014 ; Klein et al. 2014 ; Belitsky et al. 2018 ; Han et al. 2018 ).
Following the successful 1.3 mm VLBI observations in 2017, test observations at 870 μ m were conducted on the EHT array in 2018 October. Conditions at the Atacama Large Millimeter/submillimeter Array (ALMA) station during this test, including characterization of the system used there to phase the array for VLBI, are described in Crew et al. ( 2023 ). The present paper describes the VLBI test observations
2.1. Schedule
The 870 μ m fringe test observations consisted of two short scheduling blocks designed for two different subarrays. An eastern subarray, comprising ALMA, the Atacama Pathfinder EXperiment (APEX), the Greenland Telescope (GLT), the Institut de Radioastronomie Millimétrique 30 m telescope (IRAM30m), and the Northern Extended Millimeter Array (NOEMA), was scheduled to include blazar sources that were visible in the nighttime hours at all sites: CTA 102, 3C 454.3 , and BL Lac. A western subarray, comprising ALMA, APEX, GLT, and the Submillimeter Array (SMA), observed quasars J0423−0120, J0510+1800 , J0521+1638 , and J0522−3627 . The eastern subarray scheduling block was followed by several scans on BL Lac at 1.3 mm wavelength to aid diagnosis in the event of a null result. Schedule blocks for both subarrays were optimized for fringe detection at 870 μ m VLBI, and they spanned a duration of between 1 and 2 hr with at least two scans on every source. Most scans lasted 5 minutes.
The observing window consisted of five nights, 2018 October 17–21, between approximately midnight and 2:00 Coordinated Universal Time (UTC) for the eastern subarray scheduling block and between 9:00 and 11:00 UTC for the western subarray scheduling block. Each scheduling block was triggered twice within the observing window. We report herein on successful observations with the eastern array on 2018 October 18–19 and with the western array on 2018 October 21. Details of the scheduling blocks and sources observed are shown in Figure 2 .
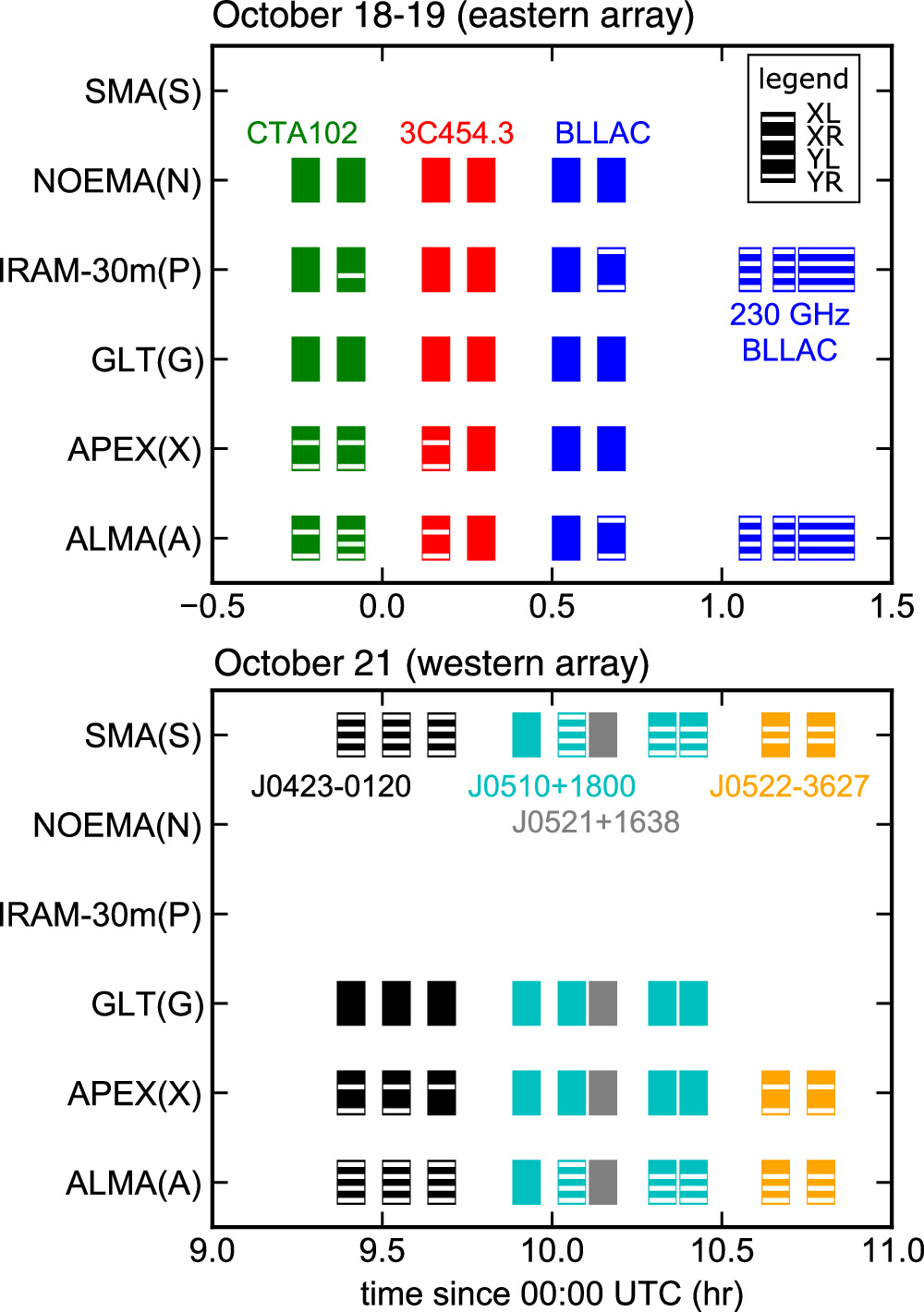
Figure 2. 870 μ m observations that yielded detections were made during two separate scheduling blocks: 2018 October 18/19 and 2018 October 21. The observations on the first night were done with an eastern array comprising ALMA, APEX, GLT, IRAM30m, and NOEMA. Observations on the second night were made with a western array: ALMA, APEX, GLT, and SMA. The scheduling blocks for both nights are shown along with the one-letter station codes, which are listed in parentheses. All detections are on baselines involving ALMA. The scans that yielded detections on baselines defined by a given station are indicated by the white horizontal ticks centered in each time block: from the top, ticks correspond to XL, XR, YL, and YR mixed polarizations per the legend at upper right. The absence of a tick indicates a nondetection. Three scans at 230 GHz (1.3 mm) were performed at the end of the eastern subarray scheduling block using just the IRAM30m and ALMA facilities.
2.2. Instrumentation and Array
Several important technologies developed for 1.3 mm VLBI are leveraged to address the challenges of 870 μ m observing, many of which are outlined in Event Horizon Telescope Collaboration et al. ( 2019b ). The VLBI backends, used to condition and digitize signals from the telescope receivers, have a cumulative data rate of 64 Gbps (Vertatschitsch et al. 2015 ; Tuccari et al. 2017 ) across four 2 GHz wide bands and two polarizations. Each station is outfitted with a hydrogen maser time standard, which had previously been found to be sufficiently stable for timekeeping in a 1.3 mm VLBI experiment and was expected to be sufficiently stable for 870 μ m.
Phased array beamforming capability is implemented at both the SMA (Young et al. 2016 ) and ALMA (Matthews et al. 2018 ) array stations. For both these stations, beamformer phasing efficiency at 870 μ m, which directly scales the visibility amplitudes measured on baselines to the station, varied from just below 50% to as high as about 80%. These efficiencies are less than what is typical for 1.3 mm (Event Horizon Telescope Collaboration et al. 2019b ). Section 3.4 has a discussion relevant to ALMA, SMA, and NOEMA 153 of phasing efficiency challenges and planned improvements to mitigate these.
The frequency setup for the 870 μ m fringe test is similar to that described in Table 4 of Event Horizon Telescope Collaboration et al. ( 2019b ). Most stations in the array observed a single 2048 MHz band at a 4–6 GHz intermediate frequency (IF) using a 342.6 GHz sky local oscillator (LO). 154 That frequency setup corresponds to a sky frequency range of 346.552–348.6 GHz. Each station observed both circular polarizations, with the exceptions of APEX (right-circular polarization only) and ALMA (dual linear, X and Y). The recorded station data were correlated using DiFX software (Deller et al. 2011 ) at the MIT Haystack Observatory. Visibility data on baselines to ALMA remained on a mixed-polarization basis (i.e., {X, Y} × {L, R}) because the observing schedules were not long enough to track polarization calibrators over a wide range of parallactic angles, which is necessary for converting the ALMA data from a linear to a circular basis (Martí-Vidal et al. 2016 ; Matthews et al. 2018 ; Goddi et al. 2019 ). Subsequent fringe fitting was done using the Haystack Observatory Postprocessing System (HOPS 155 ; Whitney et al. 2004 ; see also Blackburn et al. 2019 ).
2.2.1. ALMA
ALMA observed in dual linear polarization with IRAM-designed 870 μ m (i.e., Band 7) cartridges (Mahieu et al. 2012 ). The ALMA Phasing System (APS; Matthews et al. 2018 ) was used to aggregate the collecting area of the active dishes in the ALMA array. The APS capability had been used previously for VLBI science at 3 mm (Issaoun et al. 2019 ; Okino et al. 2022 ; Zhao et al. 2022 ) and 1.3 mm (Event Horizon Telescope Collaboration et al. 2019a , 2019b ) but not at shorter wavelengths, albeit the setup for 870 μ m observations is similar to the longer-wavelength bands. In the 870 μ m experiment, the four recorded 2.048 GHz subbands were tuned to center frequencies of 335.6, 337.541406, 347.6, and 349.6 GHz. The choice of the 337.541406 GHz frequency results from ALMA-specific tuning restrictions.
The ALMA phased array included 25 12 m antennas during the eastern track and 29 12 m antennas during the western track with a maximum antenna spacing of 600 m in both cases. Wind speeds were greater than 10 m s −1 at the ALMA site. During the eastern track, the phasing efficiency was below 50% for most of the time and at best was about 80%. During the October 21 track (western) in better weather, the phasing efficiency was more stable and greater than approximately 90% (Crew et al. 2023 ).
2.2.2. APEX
The APEX and ALMA stations are colocated, and conditions were similar at the two telescopes. APEX observed using the 345 GHz FLASH+ linear receiver (Klein et al. 2014 ). That receiver may not have been functioning optimally during the experiment and has since been replaced by the Swedish-ESO PI Instrument for APEX (Belitsky et al. 2018 ; Meledin et al. 2022 ). A quarter wave plate was used to achieve circular polarization. Two backends, a ROACH2 Digital Backend (R2DBE; Vertatschitsch et al. 2015 ) and a Digital BaseBand Converter 3 (Tuccari et al. 2017 ), were operated in parallel.
The GLT station participated in the observation but at the time was still commissioning specific subsystems. The GLT antenna has operated at Pituffik Space Base, formerly the Thule Airbase site, in Greenland since 2017 August (Inoue et al. 2014 ; Raffin et al. 2016 ; Matsushita et al. 2018 ; Koay et al. 2020 ; Chen et al. 2023 ). The GLT observed in dual linear polarization with the IRAM-made 870 μ m (i.e., Band 7) cartridges (Mahieu et al. 2012 ). The 345 GHz receiver on the GLT saw first light in continuum and spectral-line modes in 2018 August. Pointing and focus calibration at 345 GHz were still in the commissioning phase during the 870 μ m observation reported here. The GLT pointing system has since been fully commissioned for recent and future VLBI observing. Similarly, final adjustments to the dish surface had yet to be made, and the surface accuracy was estimated to be 170 μ m rms during the observations reported here. Subsequent improvements have led to rms surface accuracy in the 17–40 μ m range (see Table 7 in Chen et al. 2023 ).
2.2.4. IRAM30m
The IRAM30m telescope used the heterodyne Eight MIxer Receiver (Carter et al. 2012 ) in the 870 μ m band also known as E330. The setup and preobserving checks were analogous to a regular Global Millimeter VLBI Array or EHT session. The opacity at 870 μ m during the scheduled VLBI observations was high and would not typically have triggered single-dish science operation at this wavelength.
2.2.5. NOEMA
Portions of the NOEMA station were still being commissioned during the 870 μ m experiment. NOEMA observed in dual polarization as a single-antenna station, not as a phased array. The NOEMA receiver was a dual-polarization single-sideband unit (Chenu et al. 2016 ) with a 4 GHz bandpass. Recording was with a 16 Gbps R2DBE. The NOEMA phased array has since been commissioned for VLBI observing.
The SMA station observed with seven antennas arranged in the compact configuration with a maximum baseline of 69.1 m. The SMA Wideband Astronomical ROACH2 Machine (SWARM; Primiani et al. 2016 ; Young et al. 2016 ) was run with the VLBI beamformer mode activated, producing a coherent phased array sum of the seven antennas formatted for VLBI recording. As expected, the phasing efficiency was lower than for 1.3 mm operations. The sky LO was set to 341.6 GHz, not 342.6 GHz, to match the SWARM sky coverage with the other stations, compensating for a different IF to baseband LO because SWARM uses its own block downconverter rather than the standard EHT single-dish equipment. The data were recorded in the frequency domain at the standard SMA clock rate (4.576 Gsps), which differs from the standard EHT single-dish sample rate of 4.096 Gsps (Vertatschitsch et al. 2015 ). Adaptive Phased Array Interpolating Downsampler for SWARM (APHIDS) postprocessing was completed to interpolate and invert (from frequency domain to time domain) the SWARM data sets in preparation for VLBI correlation. After APHIDS processing, the SMA EHT data product matches that produced by the standard SMA single-dish station in sample rate and is also a time series matching the standard EHT single-dish data product.
3. Results and Discussion
Figure 1 shows that the conditions during the experiment were mixed across the array. While the observatories do not measure 870 μ m (345 GHz) opacity directly, we use MERRA-2 reanalysis and radiative transfer (Paine 2022 ) that is validated by measurements at 225 GHz (Figure 1 , black lines) to estimate τ 345 . For the eastern subarray on October 18/19, τ 345 was 0.2 at the ALMA and APEX sites and 0.8 at IRAM30m. For the western subarray on October 21, τ 345 was approximately 0.17 at the ALMA and APEX sites and 0.7 at SMA. During the experiment, the opacities at GLT and NOEMA were unfavorable, and detections on baselines to those stations were not achieved; however, both stations have weather that is compatible with 870 μ m observing and will likely yield high-frequency detections in the future (see, e.g., Raymond et al. 2021 ; Matsushita et al. 2022 ). Atmospheric conditions can change rapidly: τ 225 at the SMA decreased by nearly a factor of 4 in the hours following the experiment.
3.1. 870 μ m (345 GHz) Fringes
In VLBI, recorded data from all sites are brought to a central processing facility where data streams from each pair of sites are cross-correlated. The resulting complex correlation quantities provide a dimensionless measure of the electric field coherence between the two sites, which is proportional to a Fourier component of the brightness distribution of the target source. The correlation processor uses an a priori model to align the site data streams, recreating the exact geometry of the physical baseline connecting the two sites at the time of observation. Because the a priori model is imperfect, after processing, the cross-correlation phase typically varies as a function of time and frequency due to residual delay and delay rate, respectively. To average the correlation signal over frequency and time, the correlator output is thus searched over a range of delay and delay rate to find a peak in correlator power—a process also known as "fringe fitting" (Thompson et al. 2017 ). In this experiment, the correlator output was searched by dividing each scan into short segments and incoherently averaging them. The incoherent averaging technique (Rogers et al. 1995 ) estimates noise-debiased VLBI quantities, and it is well suited to processing low signal-to-noise ratio (S/N) VLBI data on sparse arrays as it allows integration beyond the nominal atmospheric coherence time. Figure 3 shows the dependence of amplitude in units of 10 4 and S/N on the duration of the segments for a sample scan on source J0423−0120 for the baseline comprising the ALMA and SMA stations. All four cross-hand polarizations are plotted. The scan identifier 294–0938 in Figure 3 corresponds to the day UTC for the beginning of the scan, where the day is the number of days since 2018 January 1 (294 is October 21) and UTC is the scan start time. The noise-debiased amplitude (Rogers et al. 1995 ) in Figure 3 is indicated by the horizontal blue dashed line. As the segment duration decreases, the effect of decoherence is reduced, so the S/N increases.
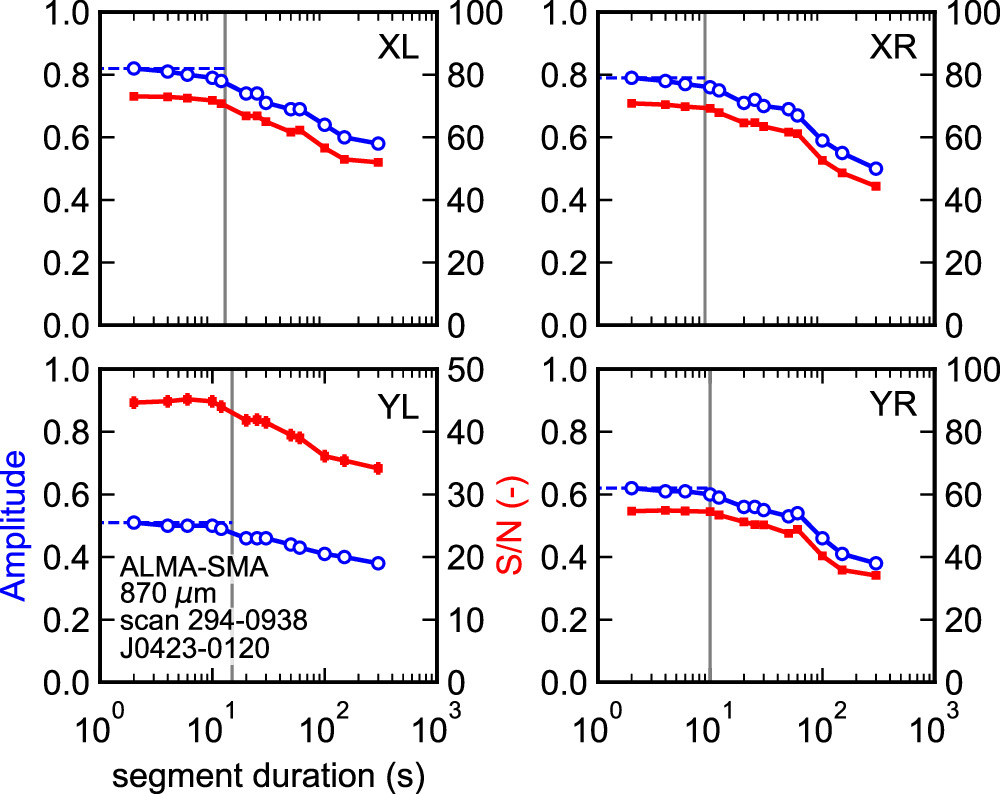
Figure 3. Scan-averaged and noise-debiased 870 μ m fringe amplitude (open blue circles, left axes) and S/N (filled red squares, right axes). Amplitudes and S/N are computed by first dividing each observing scan into short coherently integrated segments, which are then combined incoherently following the procedure in Rogers et al. ( 1995 ). Segment length is shown on the horizontal axis. Each subplot shows a different polarization on the ALMA–SMA baseline for a single scan on J0423−0120 (October 21, 09:38 UTC). Other detections listed in Table 1 have a similar dependence on segment duration but generally lower S/N. The noise-debiased amplitude and coherence time were derived using HOPS and are indicated by the horizontal blue dashed line and the vertical solid black line, respectively.
Compared to a single coherent integration over a full scan (approximately 300 s in most of the measurements), incoherently averaging the parts of a segmented scan increases the S/N by up to a factor of 2 on many of the measurements, yielding higher confidence in the detections. For most of the measurements, S/N values asymptote at the shortest segment durations. Ordinarily, we would expect the S/N values to decrease as the segments are shortened below the coherence time. The behavior we observe could be indicative of a changing coherence during the scan consistent with the windy conditions at ALMA (Crew et al. 2023 ).
Contours of fringe power versus multiband delay and rate are plotted in Figure 4 for a single scan of J0423−0120 on the ALMA–SMA baseline. The measurement exhibits a definitive peak in fringe power for each of the cross-hand polarizations. The rates are all centered near 0. Multiband delays fall within an ambiguity search window of (−8.53 ns, 8.53 ns) as they are derived from measurements spaced at ALMA's channel separation of 58.592375 MHz (Matthews et al. 2018 ; Event Horizon Telescope Collaboration et al. 2019d ).
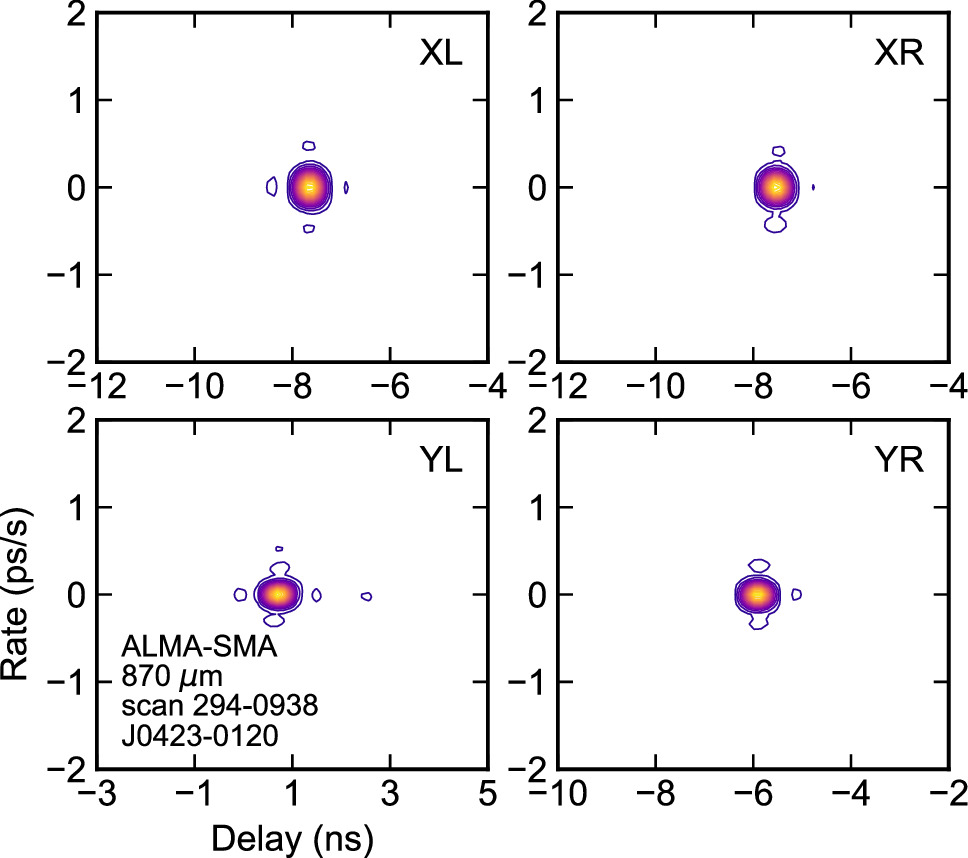
Figure 4. 870 μ m contours of incoherently averaged fringe power in 5% increments vs. delay and rate for a single scan on J0423−0120 for the ALMA–SMA baseline (October 21, 09:38 UTC). Other detections reported in Table 1 also exhibit clear peaks vs. delay/rate.
The fringe detection threshold was conservatively set at S/N > 7 to prevent false detections, and all resulting detections are summarized in Table 1 ordered by target source. The maximum spatial frequencies sampled are greater than 10.9 G λ between ALMA and the SMA, which significantly exceeds the largest spatial frequencies sampled by the EHT for M87* at 1.3 mm on the longest baseline between Hawaii and Europe (approximately 8 G λ ). The highest-S/N detections exceed 70. Simultaneous detections in all four polarization products were achieved on the ALMA–SMA baseline for J0423−0120 . The zero-baseline flux densities at 870 μ m were obtained from the ALMA local interferometry (Crew et al. 2023 ). The flux densities were 1.4, 1.0, 2.4, 1.2, and 4.9 Jy on CTA 102, BL Lac, J0423−0120 , J0510+1800 , and J0522−3627 , respectively. The source structure of the targets in this work is not known a priori, so it is not possible to say with precision how the correlated amplitudes should vary as a function of baseline length. Furthermore, these observations were designed to be a detection experiment and not carried out with all procedures that would allow robust VLBI flux density calibration. Nevertheless, the S/N on the ALMA–APEX baselines appears to be anomalously low given the short baseline length, which would ordinarily be sensitive to both small-scale structure (10–100 μ as) and larger-scale structure (10–100 mas). This is likely attributable to phase instabilities suspected in the APEX receiver (see Section 2.2.2 ), which has since been retired. Follow-on experiments, already scheduled, will focus on calibration and robust flux density measurements versus baseline length.
Table 1. 870 μ m Detections on the Indicated Baselines, Sources, and Polarizations
Baseline | Pol. | Day | Time (hh:ss) | El. 1 (deg) | El. 2 (deg) | (G ) | (s) | Delay (ns) | Rate (fs s ) | Amp. ( 10 ) | S/N |
---|---|---|---|---|---|---|---|---|---|---|---|
3C 454.3 | |||||||||||
AX | XR | 292 | 00:07 | 44.9 | 45.0 | 0.0026 | 8 | 4.4 | −1 | 0.50 | 43.7 |
AX | YR | 292 | 00:07 | 44.9 | 45.0 | 0.0026 | 8 | 5.2 | −1 | 0.47 | 41.4 |
BL Lac | |||||||||||
AP | XL | 292 | 00:38 | 24.6 | 42.6 | 9.7913 | 31 | −4.6 | 4 | 0.15 | 12.2 |
AP | YR | 292 | 00:38 | 24.6 | 42.6 | 9.7913 | 46 | −8.5 | 0 | 0.13 | 10.8 |
CTA 102 | |||||||||||
AP | YL | 291 | 23:52 | 49.7 | 43.5 | 9.9581 | 21 | 0.9 | −38 | 0.18 | 13.6 |
AX | XR | 291 | 23:44 | 48.6 | 48.7 | 0.0027 | 24 | 5.6 | −38 | 0.23 | 19.2 |
AX | XR | 291 | 23:52 | 49.7 | 49.7 | 0.0027 | 10 | 5.2 | −85 | 0.23 | 20.8 |
AX | YR | 291 | 23:44 | 48.6 | 48.7 | 0.0027 | 22 | 6.3 | −51 | 0.21 | 17.6 |
AX | YR | 291 | 23:52 | 49.7 | 49.7 | 0.0027 | 11 | 6.0 | −84 | 0.22 | 18.0 |
J0423−0120 | |||||||||||
AS | XL | 294 | 09:22 | 48.5 | 35.5 | 10.8547 | 14 | −7.6 | 6 | 0.54 | 47.8 |
AS | XL | 294 | 09:30 | 46.8 | 37.3 | 10.8874 | 14 | −8.0 | 0 | 0.70 | 62.4 |
AS | XL | 294 | 09:38 | 45.1 | 39.1 | 10.9100 | 13 | −7.7 | −2 | 0.82 | 73.1 |
AS | XR | 294 | 09:22 | 48.5 | 35.5 | 10.8547 | 9 | −7.5 | 19 | 0.60 | 53.4 |
AS | XR | 294 | 09:30 | 46.8 | 37.3 | 10.8874 | 34 | −7.9 | −0 | 0.64 | 56.6 |
AS | XR | 294 | 09:38 | 45.1 | 39.1 | 10.9100 | 9 | −7.5 | −2 | 0.79 | 70.8 |
AS | YL | 294 | 09:22 | 48.5 | 35.5 | 10.8547 | 13 | 0.8 | 19 | 0.34 | 29.6 |
AS | YL | 294 | 09:30 | 46.8 | 37.3 | 10.8874 | 17 | 0.4 | 0 | 0.47 | 41.3 |
AS | YL | 294 | 09:38 | 45.1 | 39.1 | 10.9100 | 15 | 0.7 | −2 | 0.51 | 45.2 |
AS | YR | 294 | 09:22 | 48.5 | 35.5 | 10.8547 | 10 | −5.9 | 19 | 0.46 | 40.7 |
AS | YR | 294 | 09:30 | 46.8 | 37.3 | 10.8874 | 14 | −6.3 | 0 | 0.50 | 44.2 |
AS | YR | 294 | 09:38 | 45.1 | 39.1 | 10.9100 | 10 | −5.9 | −3 | 0.62 | 54.9 |
AX | XR | 294 | 09:22 | 48.5 | 48.5 | 0.0028 | 27 | −1.0 | −8 | 0.14 | 12.6 |
AX | XR | 294 | 09:30 | 46.8 | 46.8 | 0.0028 | 39 | −0.9 | −9 | 0.16 | 13.0 |
AX | XR | 294 | 09:38 | 45.1 | 45.1 | 0.0028 | 32 | −0.9 | −11 | 0.15 | 12.9 |
AX | YR | 294 | 09:22 | 48.5 | 48.5 | 0.0028 | 30 | 0.6 | −7 | 0.14 | 10.9 |
AX | YR | 294 | 09:30 | 46.8 | 46.8 | 0.0028 | 29 | 0.7 | −9 | 0.14 | 10.8 |
J0510+1800 | |||||||||||
AS | XL | 294 | 10:01 | 37.0 | 39.6 | 10.9218 | 30 | −8.0 | −12 | 0.10 | 8.5 |
AS | XR | 294 | 10:01 | 37.0 | 39.6 | 10.9218 | 28 | −8.0 | −12 | 0.25 | 22.3 |
AS | XR | 294 | 10:17 | 34.5 | 43.4 | 10.8891 | 8 | −8.1 | −0 | 0.27 | 22.4 |
AS | XR | 294 | 10:22 | 33.5 | 44.8 | 10.8682 | 22 | 2.2 | 20 | 0.20 | 16.6 |
AS | YL | 294 | 10:01 | 37.0 | 39.6 | 10.9218 | 10 | 0.3 | −12 | 0.20 | 18.1 |
AS | YL | 294 | 10:17 | 34.5 | 43.4 | 10.8891 | 23 | 0.2 | 11 | 0.25 | 21.3 |
AS | YL | 294 | 10:22 | 33.5 | 44.8 | 10.8682 | 29 | −6.6 | 2 | 0.17 | 14.2 |
AS | YR | 294 | 10:01 | 37.0 | 39.6 | 10.9218 | 28 | −6.3 | −14 | 0.12 | 10.1 |
AS | YR | 294 | 10:17 | 34.5 | 43.4 | 10.8891 | 6 | −6.5 | 0 | 0.14 | 11.5 |
AS | YR | 294 | 10:22 | 33.5 | 44.8 | 10.8682 | 10 | 3.8 | 81 | 0.11 | 9.7 |
J0522−3627 | |||||||||||
AS | XR | 294 | 10:37 | 53.0 | 18.0 | 10.3188 | 12 | −4.7 | 38 | 0.12 | 10.1 |
AS | XR | 294 | 10:45 | 51.4 | 19.2 | 10.4084 | 24 | −4.9 | 8 | 0.20 | 12.1 |
AS | YL | 294 | 10:37 | 53.0 | 18.0 | 10.3188 | 29 | 3.5 | −4 | 0.12 | 10.3 |
AS | YL | 294 | 10:45 | 51.4 | 19.2 | 10.4084 | 22 | 3.4 | −4 | 0.16 | 14.1 |
AX | XR | 294 | 10:37 | 53.0 | 52.9 | 0.0030 | 31 | 0.8 | −1 | 0.31 | 26.9 |
AX | XR | 294 | 10:45 | 51.4 | 51.4 | 0.0030 | 39 | 0.8 | 25 | 0.25 | 15.3 |
AX | YR | 294 | 10:37 | 53.0 | 52.9 | 0.0030 | 31 | 2.3 | 1 | 0.31 | 27.0 |
AX | YR | 294 | 10:45 | 51.4 | 51.4 | 0.0030 | 31 | 2.4 | 25 | 0.29 | 24.6 |
Download table as: ASCII Typeset image
HOPS reports two coherence times: one corresponding to the point below which there is only a small amount of coherence loss within the uncertainty of amplitudes and another corresponding to the maximum S/N. For most of the scans in Table 1 , we report the former. In a few low-S/N cases where the routine was unable to fit the coherence, the coherence time based on S/N is reported instead. The coherence times across baselines range from approximately 10 to 30 s for most cases. For BL Lac, the longer coherence times may be an artifact of the moderate S/N.
3.2. 1.3 mm (230 GHz) Comparison
Presently, the EHT observes at 1.3 mm (Event Horizon Telescope Collaboration et al. 2019b ). Figure 5 compares the Fourier components of the 870 μ m detections on various sources to the 1.3 mm coverage of the 2017 EHT array on M87* (Event Horizon Telescope Collaboration et al. 2019d ). The 870 μ m detections on ALMA–IRAM30m and ALMA–SMA baselines have a higher nominal angular resolution (19 μ as) than the highest-resolution M87* detections (nominally 25 μ as).
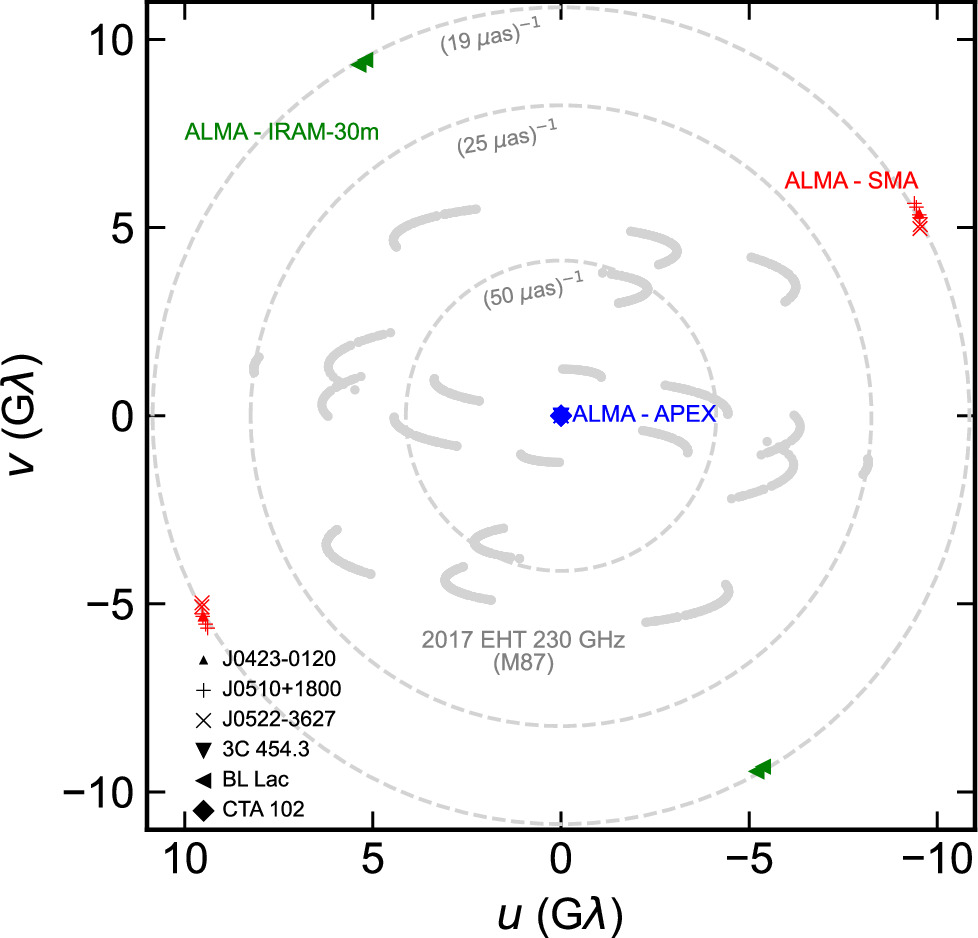
Figure 5. Detections on various targets at 345 GHz (see Table 1 ). The u – v locations of 230 GHz detections on M87* during the EHT 2017 April campaign are shown in gray including low-S/N scans at (25 μ as) −1 .
For a source-specific comparison of the 1.3 mm and 870 μ m bands, ALMA and IRAM30m observed BL Lac at 1.3 mm during three scans at the end of the eastern subarray scheduling block of the 2018 October session. Those data were searched using the same HOPS incoherent averaging method as was used for the 870 μ m observations and provide an independent application of the approach. The 1.3 mm scans provide a check of the 870 μ m processing and a point of comparison for the 870 μ m detections.
The amplitude and S/N values for one of the 1.3 mm scans are plotted in Figure 6 versus the duration of incoherently averaged segments. The S/N values are approximately tenfold greater at 1.3 mm than at 870 μ m (see Figure 3 ), which likely results from a combination of factors that boost sensitivity at the longer wavelength: lower opacity, lower receiver noise, greater aperture efficiency, a wider beam, greater coherence, and greater source flux density. The coherence time determined using HOPS was comparable for the three scans to what was found at 870 μ m: on the order of 6–30 s. As with the 870 μ m measurements, the S/N values asymptote as the segment duration decreases below the coherence time. The consistency of the S/N trends in the 870 μ m and 1.3 mm scans suggests that the behavior is a real feature of the data and not an artifact of the analysis.
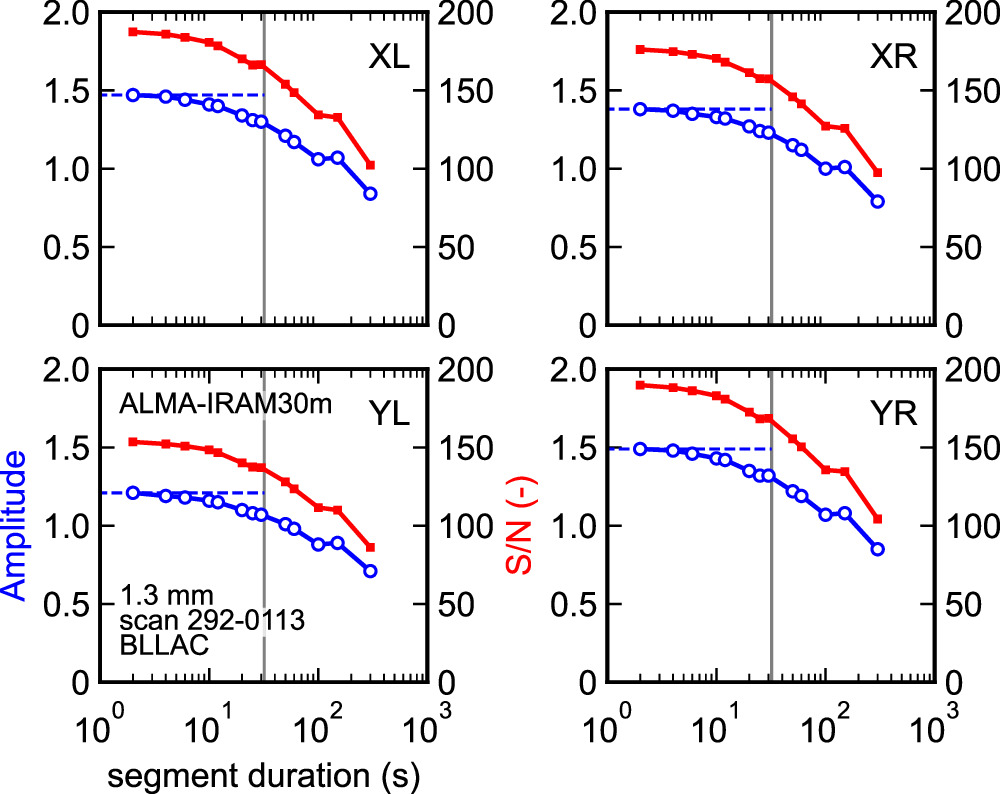
Figure 6. 1.3 mm amplitude (open blue circles, left axes) and S/N (filled red squares, right axes) vs. the duration of coherently integrated segments, which are incoherently averaged. Each subplot shows a different polarization on the baseline between ALMA and IRAM30m for a single scan on BL Lac on October 19, 01:13 UTC. Other BL Lac detections listed in Table 2 have a similar dependence on segment duration. The noise-debiased amplitude and coherence time were derived using HOPS and are indicated by the horizontal blue dashed line and the vertical solid black line, respectively. These data were calibrated in the same manner as the 870 μ m detections.
Fringe power contours at 1.3 mm are plotted as a function of multiband delay and rate in Figure 7 , exhibiting obvious peaks. The delays for each of the four polarization cross products is consistent across scans, and the 1.3 mm fringes are summarized in Table 2 . All four polarization cross-hands are detected in each of the three 1.3 mm scans. The 6.4 G λ spatial frequencies are 50% smaller than the 870 μ m scans on the AP baseline, which corresponds to the frequency scaling between the two bands. The 1.3 mm zero-baseline flux density of BL Lac deduced from the ALMA local interferometry (Crew et al. 2023 ) was 1.2 Jy.
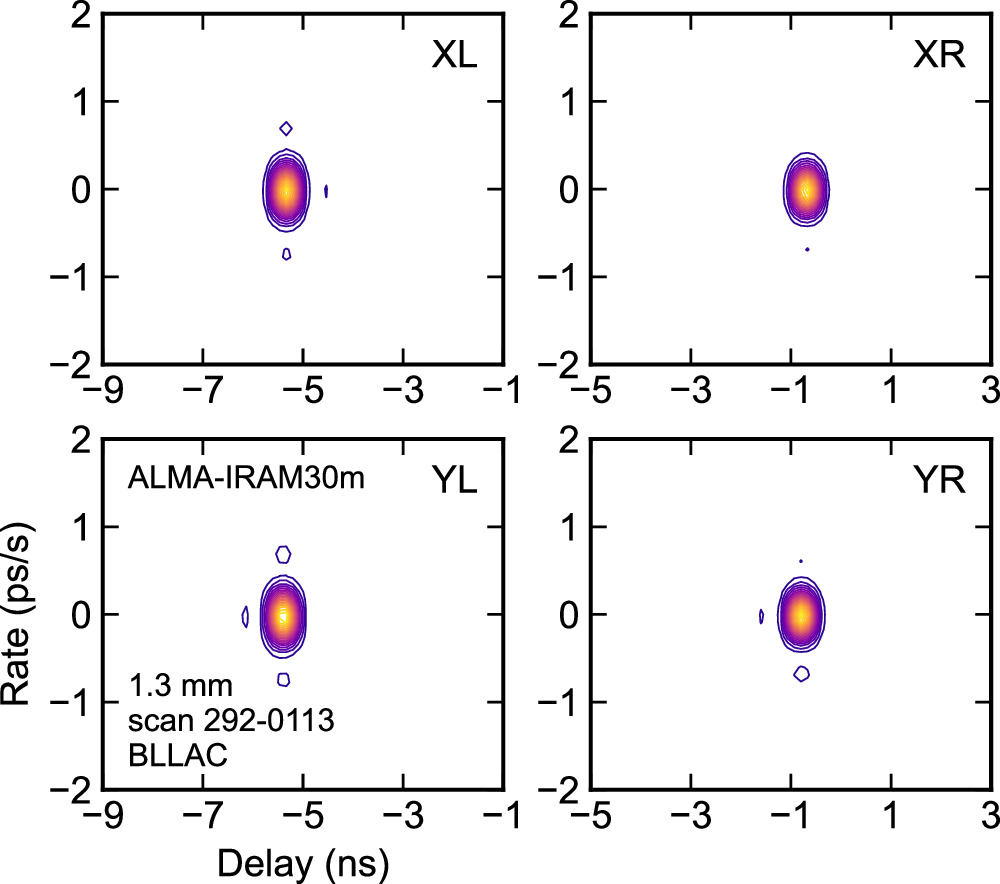
Figure 7. 1.3 mm contours of incoherently averaged fringe power in 5% increments vs. delay and rate for the baseline between ALMA and IRAM30m. This example is for a single scan on BL Lac taken on October 19, 01:13 UTC. Other detections reported in Table 2 also exhibit clear peaks vs. in delay-delay rate search space.
Table 2. 1.3 mm Detections on the ALMA–IRAM30m Baseline toward BL Lac for Indicated Polarizations
Elevation | Baseline | Delay | Rate | Amp. | S/N | |
---|---|---|---|---|---|---|
(ALMA/IRAM30m) | Length | |||||
(deg) | (G ) | (s) | (ns) | (fs s ) | ( 10 ) | |
XL | ||||||
24.5/38.3 | 6.4327 | 5 | −5.3 | −98 | 1.66 | 134.0 |
24.4/37.3 | 6.4422 | 7 | −5.3 | −66 | 1.49 | 120.0 |
24.3/36.6 | 6.4476 | 32 | −5.3 | −14 | 1.47 | 187.3 |
YR | ||||||
24.5/38.3 | 6.4327 | 6 | −0.8 | −99 | 1.77 | 143.0 |
24.4/37.3 | 6.4422 | 7 | −0.8 | −66 | 1.52 | 122.4 |
24.3/36.6 | 6.4476 | 32 | −0.8 | −14 | 1.49 | 189.8 |
XR | ||||||
24.5/38.3 | 6.4327 | 6 | −0.7 | −98 | 1.56 | 125.4 |
24.4/37.3 | 6.4422 | 7 | −0.7 | −66 | 1.37 | 110.4 |
24.3/36.6 | 6.4476 | 32 | −0.7 | −13 | 1.38 | 176.1 |
YL | ||||||
24.5/38.3 | 6.4327 | 6 | −5.4 | −98 | 1.42 | 114.4 |
24.4/37.3 | 6.4422 | 7 | −5.4 | −66 | 1.24 | 100.1 |
24.3/36.6 | 6.4476 | 32 | −5.4 | −14 | 1.21 | 153.5 |
Note. Scans listed top to bottom on October 19 begin at 01:03, 01:09, and 01:13 UTC.
3.3. Coherence and Allan Deviation
It is convenient to characterize the phase noise of an interferometer by its Allan deviation, which is a measure of fractional stability for an oscillator, time standard, or any time-variable process. When computing the Allan deviation of an observed VLBI interferometer phase, one normalizes by the frequency of observation to produce a dimensionless quantity. The relationships of Allan deviation to the statistical variance, coherence, and phase power spectrum can be found in Thompson et al. ( 2017 ). Examples of the Allan deviation of VLBI systems referenced to hydrogen maser time standards and operating at 1.3 cm and 3 mm wavelengths can be found in Rogers & Moran ( 1981 ) and Rogers et al. ( 1984 ), respectively, and show that at short wavelengths, decoherence is a potential concern. Alternatives to hydrogen masers for short-wavelength VLBI work have been explored (e.g., Doeleman et al. 2011 ). In this section, we compare the observed Allan deviation of the VLBI interferometric phase to limiting factors including the stability of time and frequency standards used in the experiment as well as instabilities due to atmospheric turbulence.
Figure 8 shows the Allan deviation for 870 μ m scans on the ALMA–SMA baseline. Over most integration times, the 870 μ m Allan deviation is comparable to but greater than the maser–maser reference. The 870 μ m traces exhibit relatively small scan-to-scan variation during the course of the brief fringe test when conditions were relatively stable. For comparison, Figure 8 also shows the Allan deviations for a large number of high-S/N 1.3 mm scans from the 2017 EHT campaign (Event Horizon Telescope Collaboration et al. 2019d ). At times of less than about 5 s, the red 1.3 mm traces all approach the limit set by the maser references. At times longer than 5 s, the red traces are noticeably scattered. The scatter exists because of the variability of atmospheric conditions during the course of an observing campaign.
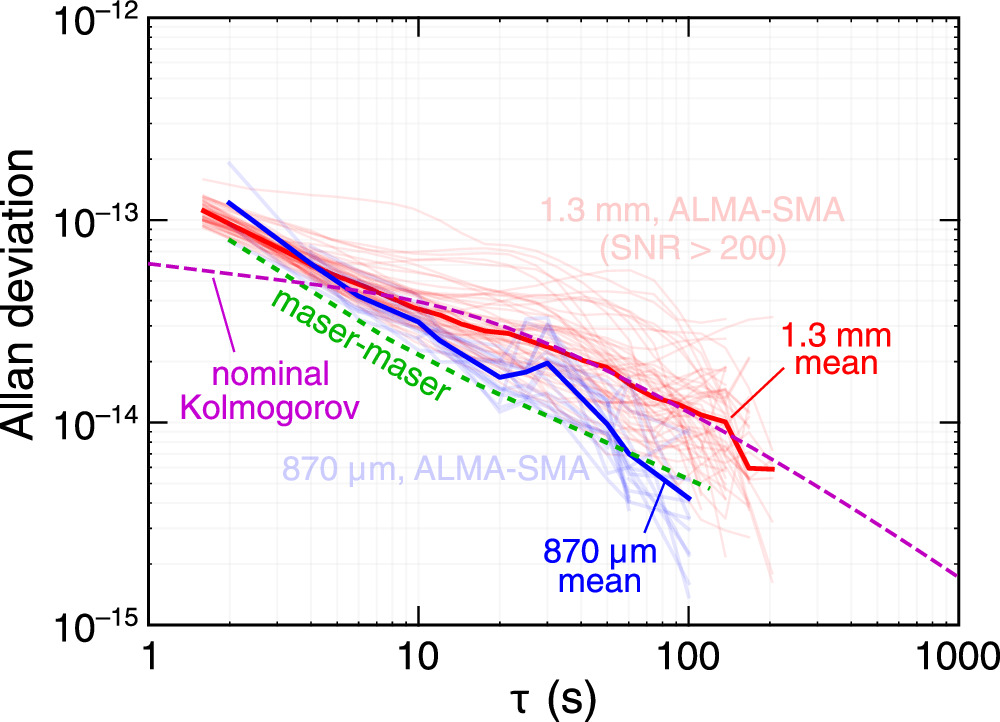
Figure 8. Allan deviation for 870 μ m (345 GHz) scans observed on the ALMA–SMA baseline (blue lines). For comparison, red lines show the Allan deviation for high-S/N scans (nominally 5 minutes long) during the 1.3 mm (230 GHz) 2017 EHT campaign (Event Horizon Telescope Collaboration et al. 2019b ). Weather variability during the 2017 campaign is responsible for the spread in those scans. The means of the individual Allan deviation traces are shown in bold for the two frequencies. The 870 μ m and 1.3 mm mean traces approach the nominal Allan deviation for a pair of T4 Science brand iMaser 3000 model masers (Thompson et al. 2017 ) at short timescales. At intermediate timescales, atmospheric turbulence can become important. The Allan deviation associated with Kolmogorov turbulence is plotted for a set of nominal parameters (Treuhaft & Lanyi 1987 ).
The tropospheric delay is essentially independent of wavelength for wavelengths longer than about 600 μ m as described by the Smith–Weintraub equation (see Thompson et al. 2017 , Chapter 13). Thus, the Allan deviation is expected to be independent of wavelength for our observations. When the atmospheric conditions are stable, the 1.3 mm Allan deviation for individual scans approaches the maser–maser limit across all integration times. The mean of the 1.3 mm scans is within a factor of approximately 2 of the mean of the 870 μ m traces. The 870 μ m mean Allan deviation on the plot happens to be lower than the 1.3 mm mean for most integration times. However, we do not consider this difference to be significant given the extremely small 870 μ m data set. Further, the observations in 2017 April and 2018 October were of course made in differing weather conditions.
To assess the impact of atmospheric turbulence at longer times, the Allan deviation associated with atmospheric Kolmogorov turbulence is plotted for a set of nominal conditions following the approach outlined by Treuhaft & Lanyi ( 1987 ): 10 m s −1 wind speed, 2 km troposphere scale height, 1.99 × 10 −7 m −1/3 Kolmogorov coefficient, and independent distant sites. The nominal Kolmogorov trace exceeds the maser–maser Allan deviation at longer times, where we expect atmospheric effects to dominate. Beyond 10 s, the nominal Kolmogorov trace matches the shape of the 1.3 mm mean. Although the 870 μ m mean falls somewhere between the maser–maser and nominal Kolmogorov limits, the atmospheric contribution may become more apparent in the future with scans spanning more variable weather conditions.
3.4. Phasing Efficiency
An important figure of merit when used to monitor the performance of phased array beamformers is phasing efficiency. This is a measure of how effectively outputs of the dishes in the local array are coherently summed to synthesize a single IF output from the array's aggregated collecting area. For each array site, periodic estimates of phasing efficiency over time are stored with other essential metadata for use in calibration.
The ALMA and SMA phased arrays experienced lower and more variable phasing efficiency during the 870 μ m test than is typical for 1.3 mm observing in similar conditions. At 870 μ m, atmospheric opacity is between 3 and 3.5 times that for 1.3 mm given the same precipitable water vapor. Further source fluxes decline with increasing frequency or shorter wavelength. Both of these factors result in a lower local array fringe S/N. There is thus greater error in the fits of the antenna phase corrections. Tuning within the band avoids the deep absorption lines due to atmospheric water resonances at 325 and 385 GHz, which would reduce the S/N still further. Also, the atmospheric phase fluctuations tracked by the adaptive phased array system have a greater amplitude for observations in the higher-frequency band. Crew et al. ( 2023 ) note that that moist, windy conditions tend to diminish phasing efficiency, and the winds were quite high at ALMA during the test. At dry, less windy times, ALMA obtained higher phasing efficiencies approaching 100%. While NOEMA participated in this test with a single dish, not as a phased array, all of these factors are expected to apply as well to NOEMA, which is now equipped with a phased array backend capable of beamforming in both the 1.3 mm and 870 μ m bands.
Water vapor radiometer (WVR) based phasing corrections were not in use during the 2018 test. Independent testing at ALMA shows that fast WVR corrections are effective at improving the efficiency when phase fluctuations are primarily due to water vapor. Phasing control loop algorithms are constantly being improved and in future will be better tuned to the 870 μ m wave band. These improvements will expand the opportunities for 870 μ m observing in a wider range of weather conditions and on weaker sources. Despite these challenges, VLBI detections at 870 μ m can be readily achieved even when phasing efficiencies are relatively low and in nonideal weather conditions.
4. Future Directions
Achieving 870 μ m VLBI fringes has strong implications for science directions that future global arrays operating at this wavelength can explore. As angular resolution scales with wavelength, we anticipate improving resolution from ∼23 to ∼15 μ as on the longest EHT baselines (Figure 5 ). Plasma propagation processes typically scale as wavelength squared, so at 870 μ m, scatter broadening of Sgr A* reduces to ∼5 μ as, further sharpening resolution and increasing signal-to-noise on the longest VLBI baselines. Similarly, Faraday rotation measured across the bandpass of EHT receivers at 870 μ m can be used to improve estimates of accretion plasma densities and magnetic field geometries close to EHT targets. For both Sgr A* and M87*, the images at 870 μ m and 1.3 mm are determined predominantly by the achromatic gravitational lensing and hence should exhibit similar characteristics, implying that the aggregate Fourier coverage of VLBI observations at different frequencies can be used to improve modeling of the gravitationally lensed emission and the imaging fidelity generally (Chael et al. 2023 ). Figure 9 shows Fourier amplitudes as a function of radius for GRMHD 156 models of M87* and Sgr A*. Inclusion of 345 GHz observations adds coverage in the visibility plane regions not sampled at 230 GHz, and it extends baseline lengths for higher angular resolution as well as enhanced overall sampling of Fourier spatial frequencies to allow dynamical reconstructions of accretion and jet launch close to the event horizon.
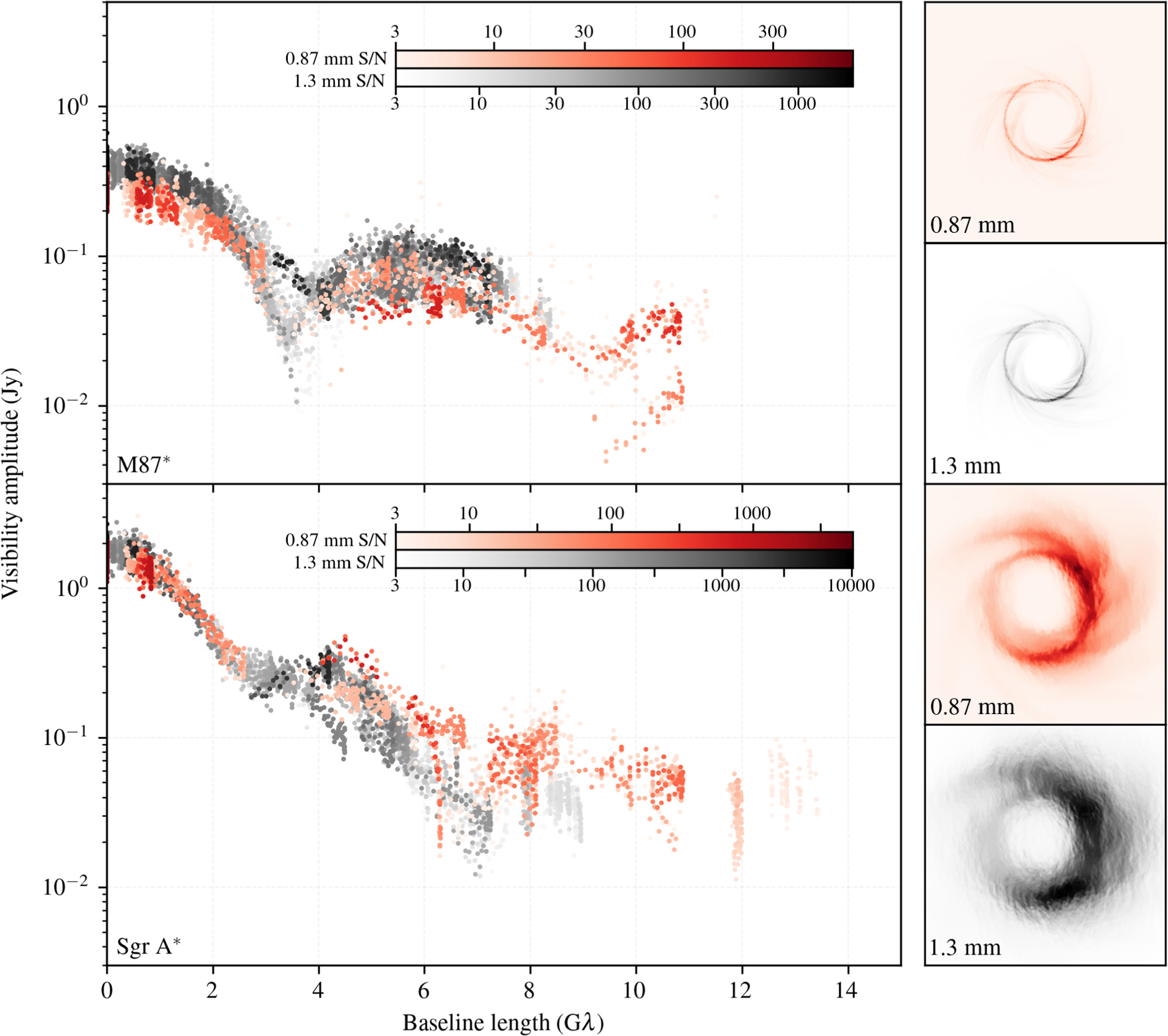
Figure 9. Left: visibility amplitudes for simulated observations of M87* (top) and Sgr A* (bottom) at observing wavelengths of 1.3 mm (gray) and 0.87 mm (red). The synthetic data have been generated using the ngehtsim package assuming array specifications appropriate for the phase 2 ngEHT array from Doeleman et al. ( 2023 ), including simultaneous dual-band observations, the use of the FPT calibration technique, and 16 GHz of bandwidth at both frequencies. Data points are colored by their S/N on an integration time of 5 minutes, and data points with S/N < 3 have been flagged. Right: images produced from GRMHD simulations of the M87* (top two panels; Event Horizon Telescope Collaboration et al. 2019e ) and Sgr A* (bottom two panels; Event Horizon Telescope Collaboration et al. 2022b ) accretion flows, used to generate the synthetic data shown in the left panels. Both simulations have been ray-traced at observing wavelengths of 1.3 mm (gray) and 0.87 mm (red), and the frequency-dependent effects of interstellar scattering have been applied to the Sgr A* images (Johnson 2016 ; Johnson et al. 2018 ).
5. Conclusions
VLBI fringe detections on baselines between ALMA–APEX, ALMA–IRAM30m, and ALMA–SMA have been achieved at 870 μ m for multiple AGN sources. S/Ns were between approximately 10 and 70. Despite marginal weather conditions across the array, detections to multiple stations and sources were obtained. This work demonstrates that the EHT instrumentation is viable at 870 μ m (345 GHz) and will provide a critical advance in array capability. EHT-wide observations at 870 μ m would yield a fringe spacing of about 15 μ as and, with a full track of coverage, would significantly enhance the fine details of the EHT images of AGN and horizon-scale targets (Doeleman et al. 2019 , 2023 ; Johnson et al. 2023 ).
Acknowledgments
This work was supported by the National Science Foundation (grants AST-1935980, AST-1743747, AST-1440254), an ALMA Cycle 5 North America Development Award, the Gordon and Betty Moore Foundation (awards GBMF3561, GBMF5278, and GBMF10423), and a generous gift from the Deepak Raghavan Family Foundation. Work on this project was conducted in part at the Black Hole Initiative at Harvard University (funded through grants 60477, 61479, and 62286 from the John Templeton Foundation and grant GBMF8273 from the Gordon and Betty Moore Foundation). This work is partly based on observations carried out with the IRAM 30 m telescope and the NOEMA Interferometer. IRAM is supported by INSU/CNRS (France), MPG (Germany), and IGN (Spain). The IRAM NOEMA phasing project was supported by the European Research Council (ERC) Synergy Grant "BlackHoleCam: Imaging the Event Horizon of Black Holes" (grant 610058). The Submillimeter Array is a joint project between the Smithsonian Astrophysical Observatory and the Academia Sinica Institute of Astronomy and Astrophysics and is funded by the Smithsonian Institution and the Academia Sinica. The SMA gratefully acknowledges the efforts of its staff for supporting these observations, including those of the operator on duty, R. Howie. This publication is based on data acquired with the APEX. APEX is a collaboration between the Max-Planck-Institut fur Radioastronomie, the European Southern Observatory, and the Onsala Space Observatory. This work was an activity external to JPL, and effort by A.R. was not in their capacity as an employee of the Jet Propulsion Laboratory, California Institute of Technology. The GLT is supported by the the Ministry of Science and Technology (MOST) of Taiwan (103-2119-M-001-010-MY2, 105-2119-M-001-042, 106-2119-M-001-013, 107-2119-M-001-041, 108-2112-M-001-048, 109-2124-M-001-005, 110-2124-M-001-007) and the National Science and Technology Council (NSTC) of Taiwan (111-2124-M-001-005, 112-2124-M-001-014).
The Event Horizon Telescope Collaboration additionally thanks the following organizations and programs: the Academia Sinica; the Academy of Finland (projects 274477, 284495, 312496, 315721); the Agencia Nacional de Investigación y Desarrollo (ANID), Chile via NCN19 058 (TITANs), Fondecyt 1221421 and BASAL FB210003; the Alexander von Humboldt Stiftung; an Alfred P. Sloan Research Fellowship; Allegro, the European ALMA Regional Centre node in the Netherlands, the NL astronomy research network NOVA, and the astronomy institutes of the University of Amsterdam, Leiden University, and Radboud University; the ALMA North America Development Fund; the Astrophysics and High Energy Physics program by MCIN (with funding from European Union NextGenerationEU, PRTR-C17I1); the Brinson Foundation; "la Caixa" Foundation (ID 100010434) through fellowship codes LCF/BQ/DI22/11940027 and LCF/BQ/DI22/11940030; Chandra DD7-18089X and TM6-17006X; the China Scholarship Council; the China Postdoctoral Science Foundation fellowships (2020M671266, 2022M712084); Consejo Nacional de Humanidades, Ciencia y Tecnología (CONAHCYT, Mexico, projects U0004-246083, U0004-259839, F0003-272050, M0037-279006, F0003-281692, 104497, 275201, 263356); the Colfuturo Scholarship; the Consejería de Economía, Conocimiento, Empresas y Universidad of the Junta de Andalucía (grant P18-FR-1769); the Consejo Superior de Investigaciones Científicas (grant 2019AEP112); the Delaney Family via the Delaney Family John A. Wheeler Chair at Perimeter Institute; Dirección General de Asuntos del Personal Académico-Universidad Nacional Autónoma de México (DGAPA-UNAM, projects IN112820 and IN108324); the Dutch Research Council (NWO) for the VICI award (grant 639.043.513), the grant OCENW.KLEIN.113, and the Dutch Black Hole Consortium (with project No. NWA 1292.19.202) of the research program the National Science Agenda; the Dutch National Supercomputers, Cartesius and Snellius (NWO grant 2021.013); the EACOA Fellowship awarded by the East Asia Core Observatories Association, which consists of the Academia Sinica Institute of Astronomy and Astrophysics, the National Astronomical Observatory of Japan, Center for Astronomical Mega-Science, Chinese Academy of Sciences, and the Korea Astronomy and Space Science Institute; the European Union Horizon 2020 research and innovation program under grant agreements RadioNet (No. 730562), M2FINDERS (No. 101018682), and FunFiCO (No. 777740); the European Research Council for the advanced grant "JETSET: Launching, propagation and emission of relativistic jets from binary mergers and across mass scales" (grant No. 884631); the European Horizon Europe staff exchange (SE) program HORIZON-MSCA-2021-SE-01 grant NewFunFiCO (No. 10108625); the Horizon ERC Grants 2021 program under grant agreement No. 101040021; the FAPESP (Fundação de Amparo á Pesquisa do Estado de São Paulo) under grant 2021/01183-8; the Fondo CAS-ANID folio CAS220010; the Generalitat Valenciana (grants APOSTD/2018/177 and ASFAE/2022/018) and GenT Program (project CIDEGENT/2018/021); the Institute for Advanced Study; the Istituto Nazionale di Fisica Nucleare (INFN) sezione di Napoli, iniziative specifiche TEONGRAV; the International Max Planck Research School for Astronomy and Astrophysics at the Universities of Bonn and Cologne; DFG research grant "Jet physics on horizon scales and beyond" (grant No. 443220636); Joint Columbia/Flatiron Postdoctoral Fellowship (research at the Flatiron Institute is supported by the Simons Foundation); the Japan Ministry of Education, Culture, Sports, Science and Technology (MEXT; grant JPMXP1020200109); the Japan Society for the Promotion of Science (JSPS) Grant-in-Aid for JSPS Research Fellowship (JP17J08829); the Joint Institute for Computational Fundamental Science, Japan; the Key Research Program of Frontier Sciences, Chinese Academy of Sciences (CAS; grants QYZDJ-SSW-SLH057, QYZDJSSW-SYS008, ZDBS-LY-SLH011); the Leverhulme Trust Early Career Research Fellowship; the Max-Planck-Gesellschaft (MPG); the Max Planck Partner Group of the MPG and the CAS; the MEXT/JSPS KAKENHI (grants 18KK0090, JP21H01137, JP18H03721, JP18K13594, 18K03709, JP19K14761, 18H01245, 25120007, 19H01943, 21H01137, 21H04488, 22H00157, 23K03453); the MICINN Research Projects PID2019-108995GB-C22, PID2022-140888NB-C22; the MIT International Science and Technology Initiatives (MISTI) Funds; the Ministry of Science and Technology (MOST) of Taiwan (105-2112-M-001-025-MY3, 106-2112-M-001-011, 106-2119-M-001-027, 106-2923-M-001-005, 107-2119-M-001-017, 107-2119-M-001-020, 107-2119-M-110-005, 107-2923-M-001-009, 108-2112-M-001-051, 108-2923-M-001-002, 109-2112-M-001-025, 109-2923-M-001-001, 110-2112-M-001-033, 110-2923-M-001-001, and 112-2112-M-003-010-MY3); the Ministry of Education (MoE) of Taiwan Yushan Young Scholar Program; the Physics Division, National Center for Theoretical Sciences of Taiwan; the National Aeronautics and Space Administration (NASA; Fermi Guest Investigator grant 80NSSC23K1508, NASA Astrophysics Theory Program grant 80NSSC20K0527, NASA NuSTAR award 80NSSC20K0645); NASA Hubble Fellowship grants HST-HF2-51431.001-A and HST-HF2-51482.001-A awarded by the Space Telescope Science Institute, which is operated by the Association of Universities for Research in Astronomy, Inc., for NASA, under contract NAS5-26555; the National Institute of Natural Sciences (NINS) of Japan; the National Key Research and Development Program of China (grants 2016YFA0400704, 2017YFA0402703, 2016YFA0400702); the National Science and Technology Council (NSTC; grants NSTC 111-2112-M-001-041, NSTC 111-2124-M-001-005, NSTC 112-2124-M-001-014); the US National Science Foundation (NSF; grants AST-0096454, AST-0352953, AST-0521233, AST-0705062, AST-0905844, AST-0922984, AST-1126433, OIA-1126433, AST-1140030, DGE-1144085, AST-1207704, AST-1207730, AST-1207752, MRI-1228509, OPP-1248097, AST-1310896, AST-1555365, AST-1614868, AST-1615796, AST-1715061, AST-1716327, AST-1726637, OISE-1743747, AST-1816420, AST-1952099, AST-2034306, AST-2205908, AST-2307887); NSF Astronomy and Astrophysics Postdoctoral Fellowship (AST-1903847); the Natural Science Foundation of China (grants 11650110427, 10625314, 11721303, 11725312, 11873028, 11933007, 11991052, 11991053, 12192220, 12192223, 12273022, 12325302, 12303021); the Natural Sciences and Engineering Research Council of Canada (NSERC; including a Discovery Grant and the NSERC Alexander Graham Bell Canada Graduate Scholarships-Doctoral Program); the National Research Foundation of Korea (the Global PhD Fellowship Grant: grants NRF-2015H1A2A1033752, the Korea Research Fellowship Program: NRF-2015H1D3A1066561, Brain Pool Program: 2019H1D3A1A01102564, Basic Research Support grant 2019R1F1A1059721, 2021R1A6A3A01086420, 2022R1C1C1005255, 2022R1F1A1075115); Netherlands Research School for Astronomy (NOVA) Virtual Institute of Accretion (VIA) postdoctoral fellowships; NOIRLab, which is managed by the Association of Universities for Research in Astronomy (AURA) under a cooperative agreement with the National Science Foundation; Onsala Space Observatory (OSO) national infrastructure for the provisioning of its facilities/observational support (OSO receives funding through the Swedish Research Council under grant 2017–00648); the Perimeter Institute for Theoretical Physics (research at Perimeter Institute is supported by the Government of Canada through the Department of Innovation, Science and Economic Development and by the Province of Ontario through the Ministry of Research, Innovation and Science); the Portuguese Foundation for Science and Technology (FCT) grants (Individual CEEC program—5th edition, https://doi.org/10.54499/UIDP/04106/2020 , PTDC/FIS-AST/3041/2020, CERN/FIS-PAR/0024/2021, 2022.04560.PTDC); the Princeton Gravity Initiative; the Spanish Ministerio de Ciencia e Innovación (grants PGC2018-098915-B-C21, AYA2016-80889-P, PID2019-108995GB-C21, PID2020-117404GB-C21); the University of Pretoria for financial aid in the provision of the new Cluster Server nodes and SuperMicro (USA) for a SEEDING GRANT approved toward these nodes in 2020; the Shanghai Municipality orientation program of basic research for international scientists (grant No. 22JC1410600); the Shanghai Pilot Program for Basic Research, Chinese Academy of Science, Shanghai Branch (JCYJ-SHFY-2021-013); the State Agency for Research of the Spanish MCIU through the "Center of Excellence Severo Ochoa" award for the Instituto de Astrofísica de Andalucía (SEV-2017–0709); the Spanish Ministry for Science and Innovation grant CEX2021-001131-S funded by MCIN/AEI/10.13039/501100011033; the Spinoza Prize SPI 78–409; the South African Research Chairs Initiative through the South African Radio Astronomy Observatory (SARAO; grant ID 77948), which is a facility of the National Research Foundation (NRF), an agency of the Department of Science and Innovation (DSI) of South Africa; the Swedish Research Council (VR); the Taplin Fellowship; the Toray Science Foundation; the UK Science and Technology Facilities Council (grant no. ST/X508329/1); the US Department of Energy (USDOE) through the Los Alamos National Laboratory (operated by Triad National Security, LLC, for the National Nuclear Security Administration of the USDOE, contract 89233218CNA000001); and the YCAA Prize Postdoctoral Fellowship.
We thank the staff at the participating observatories, correlation centers, and institutions for their enthusiastic support. This paper makes use of the following ALMA data: ADS/JAO.ALMA#2016.1.01154.V, ADS/JAO.ALMA#2011.0.00010.E, and ADS/JAO.ALMA#2011.0.00012.E. See https://almascience.nrao.edu/alma-data/eht-2018 for more detail and access to the data. ALMA is a partnership of the European Southern Observatory (ESO; Europe, representing its member states), NSF, and National Institutes of Natural Sciences of Japan, together with National Research Council (Canada), Ministry of Science and Technology (MOST; Taiwan), Academia Sinica Institute of Astronomy and Astrophysics (ASIAA; Taiwan), and Korea Astronomy and Space Science Institute (KASI; Republic of Korea), in cooperation with the Republic of Chile. The Joint ALMA Observatory is operated by ESO, Associated Universities, Inc. (AUI)/NRAO, and the National Astronomical Observatory of Japan (NAOJ). The NRAO is a facility of the NSF operated under cooperative agreement by AUI. This research used resources of the Oak Ridge Leadership Computing Facility at the Oak Ridge National Laboratory, which is supported by the Office of Science of the U.S. Department of Energy under contract No. DE-AC05-00OR22725; the ASTROVIVES FEDER infrastructure, with project code IDIFEDER-2021-086; and the computing cluster of the Shanghai VLBI correlator supported by the Special Fund for Astronomy from the Ministry of Finance in China. We also thank the Center for Computational Astrophysics, National Astronomical Observatory of Japan. This work was supported by FAPESP (Fundacao de Amparo a Pesquisa do Estado de Sao Paulo) under grant 2021/01183-8.
The EHTC has received generous donations of FPGA chips from Xilinx Inc. under the Xilinx University Program. The EHTC has benefited from technology shared under open-source license by the Collaboration for Astronomy Signal Processing and Electronics Research (CASPER). The EHT project is grateful to T4Science and Microsemi for their assistance with hydrogen masers. This research has made use of NASA's Astrophysics Data System. We gratefully acknowledge the support provided by the extended staff of the ALMA, from the inception of the ALMA Phasing Project through the observational campaigns of 2017 and 2018. We would like to thank A. Deller and W. Brisken for EHT-specific support with the use of DiFX. We acknowledge the significance that Maunakea, where the SMA EHT station is located, has for the indigenous Hawaiian people.
See, for example, Janssen et al. ( 2019 ) or the ALMA Cycle 8 2021 Technical Handbook.
NOEMA is also equipped with the phased array, though it was not commissioned at the time of this observation.
ALMA and SMA used slightly different frequency setups to match the sky frequency of the other stations; see Sections 2.2.1 and 2.2.6 .
https://www.haystack.mit.edu/tech/vlbi/hops.html
General relativistic magnetohydrodynamic.

IMAGES
COMMENTS
Find the best PhD programmes in the field of Ecology from top universities in Europe. Check all 0 programmes.
Doctoral (PhD) Positions at International Max Planck Research School - Biological Intelligence. Next application round. October 01 - November 10, 2024. Stay tuned!. The International Max Planck Research School - Biological Intelligence (IMPRS-BI) offers fully-funded doctoral student positions in the fields of behavior, ecology, neuroscience ...
Doctorates. PhD in Marine Ecology. Academic Year 2024-25. The PhD in Marine Ecology programme provides the context to develop research leading to the highest academic title of Doctor, accrediting the acquisition of skills and abilities linked to quality scientific research in the field of marine ecology, which looks at the structure and dynamics of ecosystems, the role of species and habitats ...
Completing a PhD research project in the joint University of Zurich (UZH) and Swiss Federal Institute of Technology (ETH) Zurich, PhD Program in Ecology, in one of the internationally acclaimed research groups, enhances a student's PhD experience. Students of this program are enrolled at either the UZH or the ETH. The program offers research training in the interdisciplinary field of ecology ...
Biogeochemistry and Ecosystem Ecology. Ph.D. / Full-time, Part-time / On Campus. 18,637 EUR / year. 3 years. The Open University UK Milton Keynes, England, United Kingdom. Ranked top 4%. Add to compare. Previous. 2 of 3.
9 PhD Programmes in Ecology in Europe 2024. Ecology; Overview. Individuals who are interested in the effects of climate change and environmental conservation may be interested in studying ecology at a college or university. Ecology programs concentrate their instruction on biodiversity, environmental law, forestry, and pollution in order to ...
Ecology programs concentrate their instruction on biodiversity, environmental law, forestry, and pollution in order to help students think about environmental issues on a global scale. In all, there are over 4000 Higher Education Institutions in Europe offering a wide range of courses at Bachelor, Masters and Doctorate level.
Nottingham Trent University School of Art and Design. This PhD project weaves together film, ecology, and light, by addressing light as the filmic substance in the context of ecological discourse. Read more. Supervisors: Dr L.K. Koens, Dr M Bohr. Year round applications PhD Research Project Self-Funded PhD Students Only.
24Months. Distance learning. English. Academic research for this program must provide a comprehensive study of ecology and environmental science. Read More. Featured. University of Sopron. PhD in Forestry and Wildlife Management Sciences - Roth Gyula Doctoral School. Sopron, Hungary.
The PhD in Terrestrial Ecology at the UAB offers research in global change and the loss of biodiversity, among other topics, with the support of internationally recognised scientists. ... Acces's mandates about publications, as specified on the 'Ley de la ciencia española' and the European Union's Horizon Europe.
Molecular Biosciences. Ph.D. / Full-time, Part-time / On Campus. 29,983 EUR / year. 3 years. Cardiff University Cardiff, Wales, United Kingdom. Ranked top 1%. Top 1% of Universities worldwide according to the Studyportals Meta Ranking.
We have 89 Ecology PhD Projects, Programmes & Scholarships. An Ecology PhD would give you the chance to study the relationships between organisms and their environment, through a model species, field work, or mathematical modelling. Whatever you study, from population ecology to how plants are affected by the soil ecosystem, you'll be aiming ...
Below is a list of best universities in Europe ranked based on their research performance in Ecology. A graph of 125M citations received by 4.53M academic papers made by 1,358 universities in Europe was used to calculate publications' ratings, which then were adjusted for release dates and added to final scores.
PhD opportunities. Find the right biological sciences PhD. We offer PhD degrees in ecology and evolutionary biology. Ecologists can research interactions between species and between species and their environments that influence abundance and distribution. Address climate change adaptation, design of habitat networks and agri-environment schemes.
Bournemouth University Faculty of Science & Technology. This PhD is based on developing new understandings on the ecology and conservation of brown/sea trout Salmo trutta. This is because human activities in freshwaters are driving major and unsustainable declines in freshwater biodiversity, with population reductions of over 80% since the 1970s.
Find the best PhD programmes in the field of Environmental Sciences from top universities in Europe. Check all 130 programmes. ... Ecology 45. Environmental Economics & Policy 74. Environmental Management 20. Environmental Sciences 130. ... PhD programmes in Environmental Sciences in Europe. Programmes Universities Scholarships. Page 1 | 130 ...
We are thrilled to announce a range of fully funded PhD positions available at prestigious universities throughout Europe. These exceptional opportunities span an array of cutting-edge fields, including: And more! This is your chance to be part of shaping the future of science and technology. Apply now for these fully funded PhD opportunities ...
The PhD in Marine Ecology programme provides the context to develop research leading to the highest academic title of Doctor, accrediting the acquisition of skills and abilities linked to quality scientific research in the field of marine ecology, which looks at the structure and dynamics of ecosystems, the role of species and habitats, and the relationship between biotic and antibiotic ...
PhD Student in Material Science, Physics and Environmental Sciences, University of Pannonia (Hungary) PhD Student in Analysis of Microscopic BIOMedical Images (AMBIOM), Leibniz-Institut für Analytische Wissenschaften - ISAS - e.V. (Germany) PhD Student in Compiler Technologies, NTNU Norwegian University of Science and Technology (Norway)
Professor & Chair, Graduate Program in Ecology and Evolutionary Biology, Iowa State University ... after queens stowed away in cargo ships from Europe. The new habitat suited their life cycle, ...
NOW RECRUITING: Six fully-funded PhD Scholarships at the Leverhulme Doctoral Programme for Regenerative Innovation (Regnr8-i) based in the University of Dundee. This is the first of four annual recruitment rounds inviting applications for UK Doctoral, International Doctoral and UK Master's Plus Scholarships (each for 48 to 60 months).
Ecology and Evolutionary Biology. Ph.D. / Full-time, Part-time / On Campus. 26,895 EUR / year. 3 years. University of Reading Reading, England, United Kingdom. Ranked top 1%. Top 1% of Universities worldwide according to the Studyportals Meta Ranking.
A Rutgers-led study shows diminished crop yield is globally common but low yields could be addressed by increasing the number of pollinators . A team of researchers led by Rutgers University-New Brunswick scientists has analyzed crop yields of more than 1,500 fields on six continents, and found that production worldwide of important, nutritionally dense foods such as fruits, vegetables, nuts ...
The American Astronomical Society (AAS), established in 1899 and based in Washington, DC, is the major organization of professional astronomers in North America.Its membership of about 7,000 individuals also includes physicists, mathematicians, geologists, engineers, and others whose research and educational interests lie within the broad spectrum of subjects comprising contemporary astronomy.
1 of 20. Find the best PhD programmes in the field of Environmental Studies & Earth Sciences from top universities in Europe. Check all 392 programmes.
Choose from a wide range of doctoral programmes that will take your academic, scientific, and research career further. Masaryk University is one of Central Europe's most prestigious and respected higher education institutions. With over 31,000 students, it is also the second-largest university in the Czech Republic.
Bournemouth University Faculty of Science & Technology. This PhD is based on developing new understandings on the ecology and conservation of brown/sea trout Salmo trutta. This is because human activities in freshwaters are driving major and unsustainable declines in freshwater biodiversity, with population reductions of over 80% since the 1970s.